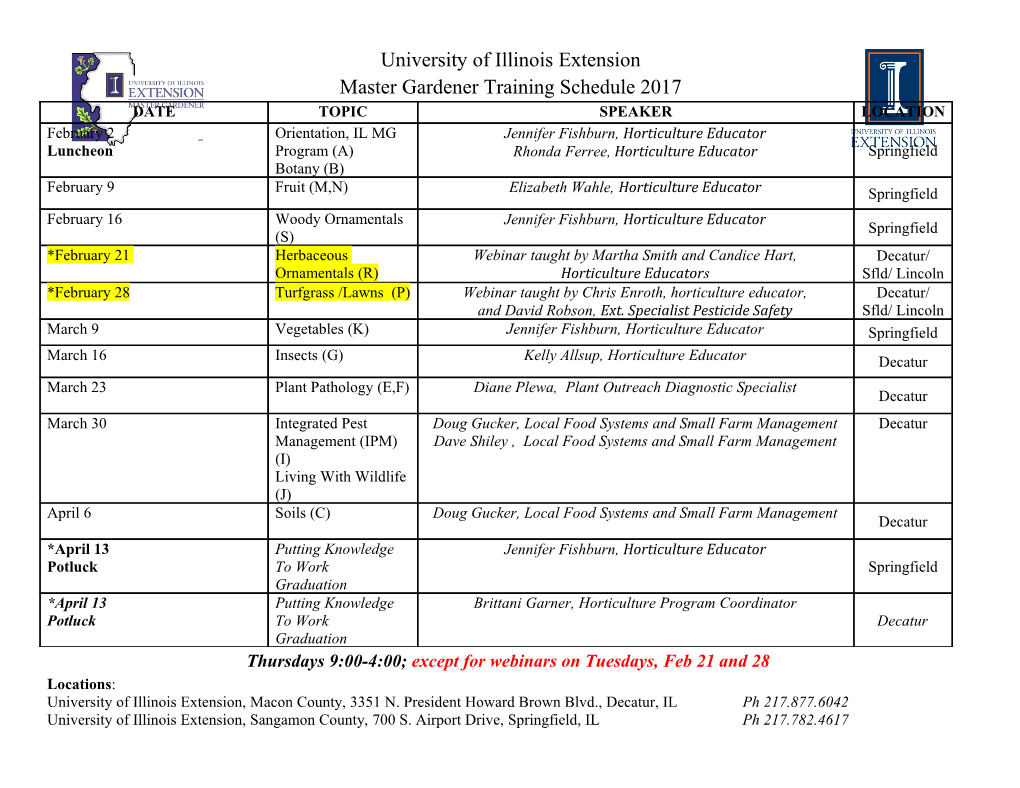
Nitroxyl-Mediated Peroxide Modification of Polypropylene by Benjamin Rhys Jones A thesis submitted to the Department of Chemical Engineering In conformity with the requirements for the degree of Master of Applied Science Queen’s University Kingston, Ontario, Canada (May 2021) Copyright ©Benjamin Rhys Jones, 2021 Abstract The thermal stability of alkoxyamine derivatives of polypropylene (PP) is examined through studies of model hydrocarbon systems and atactic-PP substrates. Primary, secondary and tertiary alkoxyamines are integral to modern techniques for modifying the architecture of linear PP materials, and detailed knowledge of their stability at polymer processing temperatures is needed to further understand the underlying principles of nitroxyl-based formulations. GC analysis of alkoxyamines derived from 2,4-dimethylpentane + TEMPO show that the tertiary regioisomer is susceptible to disproportionation to alkene + HOTEMPO at temperatures as low as 140 oC. Extension of these thermolysis experiments to atactic PP-g-HOTEMPO mirrored the model compound results, with appreciable extents of nitroxyl generation observed over a 20 min timescale at 180 oC. This reaction extent is not expected to dramatically alter the performance of nitroxyl-based formulations that modify the structure and composition of PP homopolymers. The yield of H-atom abstraction by peroxide-derived alkoxy radicals is another fundamental reaction that underlies polyolefin modifications. Indirect measures based on analysis of peroxide byproducts are supplemented with a fluorescence spectroscopy method that quantifies polymer- bound alkoxyamines. H-atom transfer from high density polyethylene (HDPE) and poly(ethylene-co-propylene) (EPR) was gained by using napthoyloxy-TEMPO, whose fluorophore supports a highly sensitive analytical method. This technique is extended to studies of reactive HDPE/EPR blending to ascertain the extent of peroxide migration from one phase to the other during the compounding process. The data indicates that mass transfer of the peroxide is competitive with the rate of peroxide breakdown, causing both polymers to incur structure modification irrespective of how the initiator is charged to the blend components. i Acknowledgements I would like to thank Dr. Scott Parent for his expertise and guidance through this new field of study for me. I would like to thank my lab mates: Mitch Sadler, Kyle Ford and Matt Hawrylow for making my time in the lab more enjoyable. I’d also like to thank all the other staff and students in the department who made these times memorable. Special shoutout to Andrew Sellathurai for keeping me sane during the COVID-19 pandemic. Lastly, I would like to thank my partner, Kylie, and my family, who were always there to support me. To my mother, who always encouraged me to continue my education, while only encouraging my brother, Craig, to eat more bacon. This is for you. ii Table of Contents Abstract…………………………………………………………………………………………….i Acknowledgements………………………………………………………………………………..ii List of Figures…………………………………………………………………………………….vi List of Tables…………………………………………………………………………………….vii List of Schemes…………………………………………………………………………………viii List of Equations………………………………………………………………………………….ix List of Abbreviations……………………………………………………………………………...x Chapter 1 Introduction………………………………………………………………………….…1 1.1 Polypropylene Degradation………..……………………………………………….....1 1.2 H-Atom Abstraction by Peroxide Initiators...………………………………………....2 1.3 Nitroxyl Trapping of Alkyl Radicals………………………..………………………...4 1.4 Alkoxyamine Instability………………………………..……………………………...7 1.5 Model Compounds…………………………………………………..………………...8 Chapter 2 Experimental Methods………………………………………………………………..10 2.1 Materials………………………………………………………………..…………....10 2.2 Instrumentation and Analysis……………………………..…………………………11 2.3 Synthesis of 1-methoxy-2,2,6,6-tetramethyl-1-piperidine (Me-TEMPO)…………...12 2.4 Synthesis of (2,2,6,6,-tetramethylpiperidine-N-oxyl)-2,4-dimethylpentane regioisomers (DMP-TEMPO isomers)……………………………………...…………...12 2.5 Synthesis of 1-(2,2,6,6-tetramethylpiperdine-N-oxyl)-cyclohexane (cyclohexane-g-TEMPO)...................................................................................................13 2.6 Thermolysis of cyclohexane and DMP alkoxyamines…………………………...…..14 iii 2.7 Synthesis of PPatactic-g-HOTEMPO…………………………………………..……...14 2.8 Thermolysis of PPatactic-g-HOTEMPO…………………………………………….....14 2.9 Synthesis of Model Compounds for AE Determinations……………………………15 2.10 Synthesis of 4-(1-Naphthoyloxy)-2,2,6,6-tetramethylpiperdine-1-oxyl (N- TEMPO)……………………………………………………………………………….....15 2.11 Synthesis of Dodecyloxy-(4-(1-Naphthoyloxy)-2,2,6,6-tetramethylpiperdine) (Dodecane-NTEMPO)……………………………………………………………….…..16 2.12 Synthesis of Polymer-g-NTEMPO samples (EPR/HDPE-g-NTEMPO)………...…17 2.13 Synthesis of EPR/HDPE-g-NTEMPO Polymer Mix……………………………….18 Chapter 3 –Tertiary Alkoxyamine Disproportionation…………………………………………..19 3.1 Introduction…………………………………………………………………..………19 3.2 Results and Discussion………………………………………………………..……..20 3.3 Conclusion………………………………………………………………………..….26 Chapter 4- Fluorescence Determination of Macroradical Yields in Polyolefin Melt Blends……………………………………………………………………………………………28 4.1 Introduction………………………………………………………………………..…28 4.2 Results and Discussion…………………………………………………………..…..31 4.2.1 Abstraction Efficiency Validations………………………………….…..…31 4.2.2 Independent HDPE and EPR Modifications……………………………….33 4.2.3 HDPE + EPR Blend Modifications…………………………….……….....38 4.3 Conclusion………………………………………………………………………..….41 Chapter 5 Future Work…………………………………………………………………………..42 5.1 Tertiary Alkoxyamine Instability…………………………..………………………………...42 iv 5.2 Peroxide Migration Trials………………………..…………………………………………..42 References………………………………………………………………………………………..42 Appendix…………………………………………………………………………………………49 Appendix A 1H NMR spectrum of dodecane-g-NTEMPO……………………………...49 Appendix B 1H NMR of PP-g-TEMPO………………………………………………….50 v List of Figures Figure 1. GC signal integrations from nitroxyl liberated by Cyclohexane-g-NTEMPO and DMP-g-NTEMPO at 140oC………………………………………………………………...……22 Figure 2. GC signal integrations from nitroxyl liberated by Cyclohexane-g-NTEMPO and DMP-g-NTEMPO after 20 min …………………………………………………………………23 Figure 3 H-atom abstraction efficiency measurements acquired with different analytical techniques………………………………………………………………………………………..33 Figure 4 Calibrations of dodecane-g-NTEMPO in cyclohexane (295nm excitation, 280-580nm emission at 20oc) and dodecane-g-NTEMPO in xylenes (295nm excitation, 315-430nm at 125oc) ……………………………………………………………………………………………35 Figure 5. Comparison of dodecane-g-NTEMPO and EPR-g-NTEMPO fluorescence spectra in cyclohexane (20oC, 295 excitation wavelength)..……………………………………………..36 Figure 6 Comparison of HDPE-g-NTEMPO and dodecane-g-NTEMPO fluorescence spectra in xylenes (125oC, 295 excitation wavelength)..………………………………………………...37 Figure 7. EPR-g-NTEMPO and HDPE-g-NTEMPO alkoxyamine yields……………………...38 Figure 8. Fluorescence spectra for EPR-g-NTEMPO isolated from the compounded blend and its parent material …………………………………………………………………………...40 Figure 9. Fluorescence spectra for HDPE-g-NTEMPO isolated from the compounded blend and its parent material……………………………………………………………………………40 vi List of Tables Table 1. Amount of nitroxyl liberated from PP-g-HOTEMPO at 140oC…………………….....25 Table 2. Amount of nitroxyl liberated from PP-g-HOTEMPO after 20 min …………………...25 Table 3. AE of DCP acting upon various model hydrocarbons at 160oC……………………….32 vii List of Schemes Scheme 1. Production and -scission of tertiary macroradicals …………………………………1 Scheme 2. Peroxide thermolysis and hydrogen abstraction……………………………………….2 Scheme 3. Nitroxyl trapping of alkyl radicals produced by peroxide initiator……………………5 Scheme 4. Disproportionation products of 4-TEMPO-2,4,6-trimethyl heptane…………………..8 Scheme 5. Model alkoxyamine studies…………………………………………………………..20 Scheme 6: Cumyloxyl scission and H-atom abstraction products of AE determinations……….29 Scheme 7. Structure of NTEMPO……………………………………………………………….30 viii List of Equations Equation 1. Abstraction Efficiency………………………………………………………………..3 Equation 2. Trapping Ratio………………………………………………………………………..5 Equation 3. Induction time provided by a nitroxyl formulation…………………………………..6 ix List of Abbreviations 1o – primary 2o - secondary 3o – tertiary AE – abstraction efficiency BDE – bond dissociation energy CDCl3 – deuterated chloroform DCP – dicumyl peroxide DMP – 2,4-dimethylpentane DMP-TEMPO – (2,2,6,6-tetramethylpiperidine) 2,4-dimethylpentane isomers DMSO – dimethylsulfoxide EPR – ethylene propylene rubber . FeSO4 7H2O – iron (II) sulfate heptahydrate g – gram GC – gas chromatography H2O – water H2O2 – hydrogen peroxide H2 – hydrogen HOTEMPO – 4-hydroxy-2,2,6,6-tetramethylpiperdine-N-oxyl HDPE – high density polyethylene k – rate constant L130 – 2,5-di(tert-butylperoxy)-2,5-dimethyl-3-hexyne L231 – 1,1-bis(tert-butylperoxy)-3,3,5-trimethylcyclohexane Me-TEMPO - 1-methoxy-2,2,6,6-tetramethyl-1-piperidine MW – molecular weight N2 – nitrogen NaSO4 – sodium sulfate NMR – nuclear magnetic resonance x NTEMPO – 4-(1-Naphthoyloxy)-2,2,6,6-tetramethylpiperdine-1-oxyl Min – minutes MS – mass spectroscopy PE – polyethylene PIB – polyisobutylene PP – polypropylene ppm – parts per million t1/2 – initiator half lives tind – induction time TEMPO – 2,2,6,6-tetramethylpiperidine-N-oxyl TMIO - 1,1,3,3-Tetramethylisoindolin-2-yloxy TPE – Thermoplastic Elastomer TPV – Thermoplastic Vulcanizate VTES – vinyl triethoxy silane xi Chapter 1 Introduction 1.1 Polypropylene Degradation The mechanism
Details
-
File Typepdf
-
Upload Time-
-
Content LanguagesEnglish
-
Upload UserAnonymous/Not logged-in
-
File Pages62 Page
-
File Size-