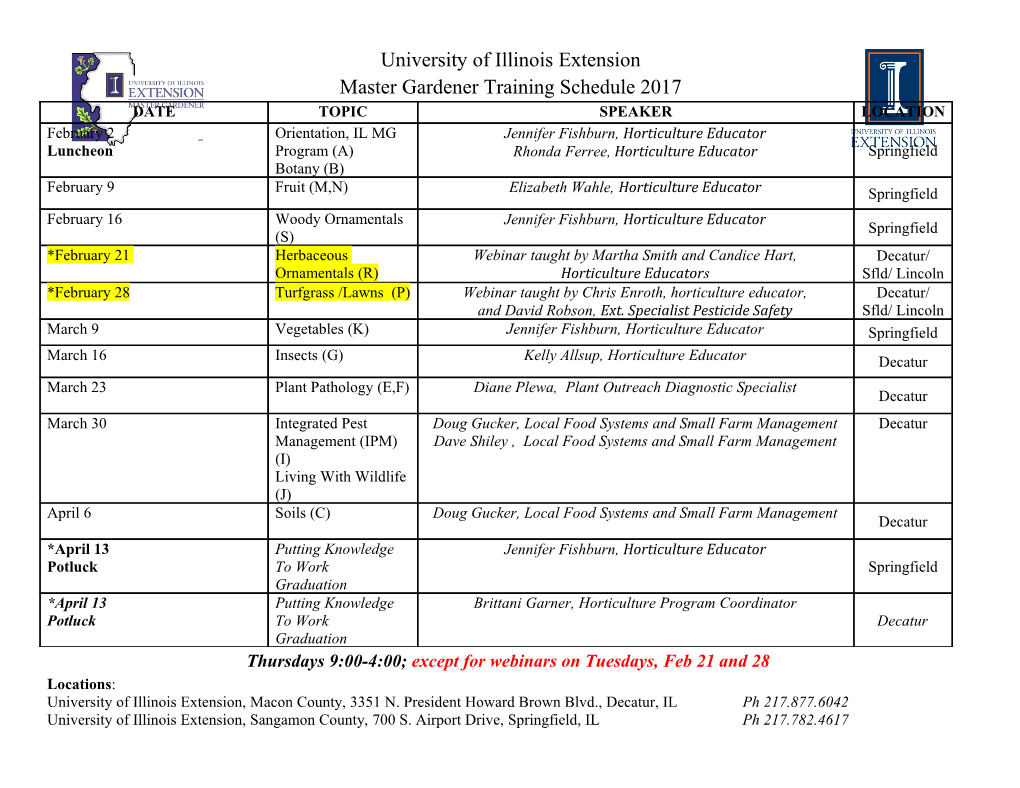
MonitoringMonitoring ElEl NiñoNiño WithWith SatelliteSatellite AltimetryAltimetry Dr. Don P. Chambers Center for Space Research The University of Texas at Austin El Niño Workshop- Online for Education March 16, 1998 Center for Space Research, The University of Texas at Austin Introduction El Niño has received a lot of attention this year, mostly due to the fact that several different instruments, both in the ocean and in space, detected signals of an impending El Niño nearly a year before the warming peaked. One of these instruments was a radar altimeter on a spacecraft flying nearly 1300 km (780 mi.) over the ocean called TOPEX/Poseidon (T/P). T/P was launched in September 1992 and is a joint mission between the United States and France. It is managed by NASA’s Jet Propulsion Laboratory and the Centre National d’Etudes Spatiales (CNES). In this presentation, I will describe in general the altimeter measurement and discuss how it can be used to monitor El Niño. In particular, I will discuss how the altimetry signal is related to sea surface temperature, and how it is different, and how altimetry can detect El Niño signals months before peak warming occurs in the eastern Pacific. To begin with, I would like to show two recent images of the sea-level measured by TOPEX/Poseidon and point out the El Niño signatures. Then, I will discuss the measurements that went into the image and discuss how we know they are really measuring what we think they are. This is the sea-level anomaly map from the TOPEX/Poseidon altimeter, for January 28 to February 7, 1998. This is the sea-level anomaly map from the TOPEX/Poseidon altimeter, for February 7 to February 17, 1998. Notice the changes occuring in the eastern Pacific. A sea-level anomaly is the difference between the total sea-level and an average sea-level for this time of year. We look at anomalies because the total sea- level measurement made by the altimeter varies from ± 100 meters. Most of this is constant, though, and is due to the Earth’s gravity and the ocean circulation. Sea- level variations caused by El Niño account for less than 1% of the total signal. If the constant part were not removed, the El Niño signal would not be observable. El Niño is evident in the figures on the previous page as higher than normal sea- levels in the east, and lower than normal sea-levels in the west. Sea-level is beginning to drop in the east, though, and indications are that El Niño is beginning to dissipate. The sea-level is actually highest north and south of the equator. This is a sign that El Niño is dissipating, and will be discussed later. Also, note the high sea- levels in the Indian Ocean, which are nearly a mirror-image to El Niño in the Pacific. I will discuss this later in the presentation as well. The altimeter does not directly produce the image shown on the previous slide. Instead, it measures the height of the spacecraft above the ocean surface, which can be converted into sea-level. These sea-level measurements can then be mapped to a uniform grid, color coded, and displayed. However, the conversion to the sea-level measurement is not a simple procedure. Recall that TOPEX/Poseidon orbits the Earth at an altitude of 1300 km. The altimeter sends several thousand radar pulses towards the ocean each second then measures the time for the pulse to return to the spacecraft. Not only does the computer have to match the right transmitted pulse with the right received pulse, but it has to compute the time of transit properly. An error in time of less than a second would mean an error of tens of centimeters. To get the height of the satellite above the ocean, the time for the pulse to return has to be converted to range using the speed of light. However, the speed of light is constant only in a vacuum. The radar pulse travels through the atmosphere twice, where it is refracted by air molecules, water vapor, free electrons, and is partially scattered by surface waves. The size of these errors add up to more than 3 meters. However, all of them can be measured or modeled. Finally, the precise location of the satellite needs to be known, since the sea- level is the difference between the satellite location above the center of the Earth and the height of the satellite above the ocean. If the satellite location were only good to 1 meter, then the sea-level measurement would only be good to 1 meter. But, how accurate is the image on the previous slide? The accuracy is amazing, considering all of these problems listed above: about 2 to 3 cm (1 in). That’s about the diameter of a quarter. We know this because we can compare sea-level measured by T/P with sea-level measured at the ocean surface with tide gauges. Here we have compared altimeter measurements with nearby tide gauges at two sites: Pohnpei in the western Pacific and Baltra in the eastern Pacific. Sea-level in these regions are very large during El Niño events (indicated by arrows). Sea-level is much lower than normal in the west and higher than normal in the east as will be explained later. If the tide gauges are considered to be perfect (which they are not), the accuracy of the altimeter is about 3 cm. However, if a more realistic error is assumed for the tide gauges then the accuracy of the altimeter measurement is around 2 cm. Note that we are using the mean standard deviation as a measure of error; differences at some times can be up to 3 times this number. Also, comparisons at some tide gauges are better or worse. The average is about 2.5 cm. Pohnpei in Western Pacific Baltra in Eastern Pacific (7°N, 158°E) (0°, 90°W) Standard Deviation of Differences = 3 cm Standard Deviation of Differences = 3.5 cm 20 30 T/P 10 20 Tide Gauge 0 10 -10 0 -20 T/P -10 Tide Gauge Sea-Level Anomaly (cm) Sea-Level Anomaly (cm) -30 -20 1993 1994 1995 1996 1997 1998 1993 1994 1995 1996 1997 1998 Year Year Tide Gauge TAO Moored Buoy T/P Groundtrack 30.0 20.0 10.0 0.0 latitude (°N) -10.0 -20.0 -30.0 50 100 150 200 250 300 longitude (°E) T/P flies in a groundtrack that repeats 30 every 10-days and goes as far north and 20 south as 66° latitude. This means that it samples approximately 400000 points over 10 the ocean every 10-days. Compare that to 0 ship measurements, moored buoys, or tide Latitude (°N) -10 gauges. However, there are still gaps in the data, which means that the image in the -20 earlier slide is based on interpolating real -30 measurements to a constant grid, then 40 50 60 70 80 90 100 110 120 smoothing them and plotting them Longitude (°E) graphically and assigning colors based on Lines are T/P groundtrack and dots the heights. are locations with more than 24 ship measurements in 3 years. Before describing how altimetry can monitor El Niño, I would like to discuss how El Niño begins, and why El Niño warming causes a sea-level variation. During normal conditions, winds blow from east to west, due to differences in the atmospheric pressure. Normally, a high pressure system sits over the eastern Pacific, while a low pressure system sits over the western Pacific. Because of the low pressure system in the west, there is increased upward convection which puts water vapor into the atmosphere and results in more rainfall in the west than in the east. At the same time, the surface currents along the equator generally move east to west. This transports water warmed year round by the sun to the western Pacific, where it tends to pile up before flowing north and south as other currents. This pushes down the thermocline, or the region where the temperature change with depth is the greatest. In the east, cold water upwells, or rises up from great depths to replace the warm water which flowed west, and the thermocline is shallow. All of this will cause a certain sea-level signature, since sea-level is a measure of the integrated water density. Warmer water has a lower density than colder water, and takes up a greater volume. Thus, sea-level is higher where the thermocline is depressed and the upper waters are warm, and sea-level is lower where the thermocline is raised and upper water is cool. Changes in the atmosphere over the western Pacific cause all of this to change. Figure courtesy of NOAA PMEL Several weeks to several months before El Niño begins to manifest in the eastern Pacific, a dramatic change occurs in the atmosphere over the Pacific. The pressure over the western Pacific increases while the pressure in the east decreases. This causes a change in the wind pattern and the convection, as shown in the figure below. This atmospheric change is called the Southern Oscillation. The Trade Winds decrease or even reverse and blow from west to east. The equatorial surface current slows, and a subsurface current that always exists strengthens. This undercurrent has a core at about 100 meters depth and always flows west to east. Most of the time it dies out in the central Pacific. During El Niño, though, the current is strong all the way to the Galapagos Islands off the coast of South America; it sometimes surfaces.
Details
-
File Typepdf
-
Upload Time-
-
Content LanguagesEnglish
-
Upload UserAnonymous/Not logged-in
-
File Pages27 Page
-
File Size-