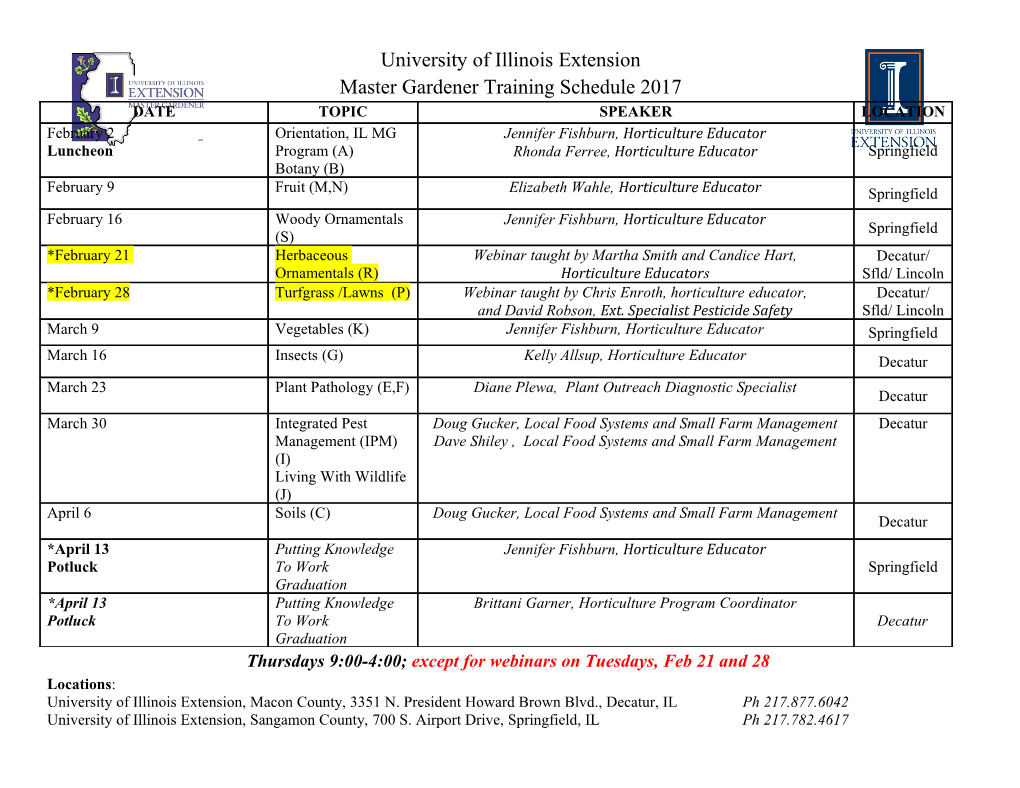
Bioengineering The Fracture Callus: Bone Repair Through Fracture Mimetics By Wollis Jude Vas Thesis submitted in fulfilment of the requirements for the degree of Doctor of Philosophy in the Division of Surgery and Interventional Science at University College London. November 2018 1 Acknowledgements Firstly, I would like to thank Dr Scott Roberts for providing me with the opportunity to peruse my PhD. His extensive knowledge of bone biology and tissue engineering has helped guide and shape this project, without whom this project would not be possible. I would also like to thank ORUK for providing me with the funding that supported my PhD programme and made this work possible. The members of the research group have also made massive contributions to the work being presented in this thesis, I would, especially, like to thank Dr Mittal Shah for supporting and guiding me through many of the challenges I faced. Dr Umber Cheema has also ensured that I experienced the full benefits of being the student at the division. Overall, I would like to extend my gratitude to the members of IOMS, who were there to show me the ropes as I started my journey as a PhD student. I would like to thank Prof Frank Luyten for his contribution of human periosteal stem cells, used extensively throughout my work. I would also like to thank Prof Duchen and Dr Blacker for lending me their support and expertise with the SHG/FLIM imaging. I would like to thank again the Co-Authors Dr Mittal Shah, Rawiya Al Hosni, Dr Helen C Owen and Dr Scott Roberts who contributed to my published literature review which was the basis for the introduction to my thesis. Ultimately, all this work would not be possible without the unconditional support I received from my family. They have joined me in celebrating my milestones while being ever present to support me during my troubles. 2 Abstract Fracture non-union is estimated to occur in approximately 5-10% of bone fractures. Tissue engineering strategies that aim to replicate mature tissue may be unresponsive to the cues observed during the initiation of fracture repair, and as such have limited ability to integrate with the host tissues. As such, this thesis aims to develop an implant that mimics the cartilaginous callus, the initial reparative stage of the body’s response to fracture; through the development of decellularised xenogeneic hyaline cartilage in combination with skeletal (stem) cells. This thesis presents data on the optimisation of an osmotic shock based decellularisation methodology (Vac-OS) for costal cartilage. The resultant scaffolds (dcECM) were investigated for the removal of cellular content and maintenance of cartilage-specific proteins such as sulfated glycosaminoglycans. The Vac-OS methodology efficiently lowered DNA content to levels below in vivo immunological response thresholds, while eliminating the highly immunogenic and abundant alpha-gal epitope. Interestingly, immunogenic neutralisation was achieved while retaining over 80% of the sGAG content, surpassing published hyaline cartilage decellularisation methodologies. Extracellular matrix (ECM) integrity was using a novel methodology based on fluorescence lifetime imaging (FLIM) and further assessed using differential scanning calorimetry (DSC). Both FLIM and DSC indicated a lack of significant change in cartilage ECM integrity, post Vac- OS decellularisation. The bioactivity of the dcECM was subsequently assessed using various skeletal cell populations, indicating an inherent capacity to enhance chondrogenesis. Furthermore, post-implantation in immunocompetent mice, the dcECM promoted a regenerative response, in contrast with native costal cartilage. The dcECM was also fabricated into porous scaffolds capable of being upscale to meet clinical demands and gel coatings that enhance in vitro chondrogenesis. This thesis demonstrates the dcECM’s potential as a fracture callus mimetic. The study, therefore, concludes that these constructs could potentially enhance bone repair in cases of atrophic non-union fracture, where the failure of callus formation is a defining event. 3 Impact Statement Fracture non-union results in around 10% of all fractures. Currently, the gold- standard treatment for non-unions remains autologous bone grafts (ABG). The use if ABG, however, requires multiple operations and is often accompanied by reports of complication such as post-operative pain, and is ultimately limited by the amount of available donor tissue. Moreover, synthetic and natural bone grafts substitutes attempt to mimic the characteristics of autologous bone grafts are often unresponsive to the cues present at early stages of endochondral fracture healing, resulting in poor graft remodelling and integration into host tissue. The novel research carried out during the duration of this project focused on developing a grafting material that mimicked the early phase of fracture repair; The fracture callus. This approach harnessed the recent evidence generated by the scientific community that has driven a gradual shift towards engineering a developmental or repair process to produce more biomimetic strategies for bone repair. In 2017, an article was published in Tissue Engineering: Part A, highlighting the creation of a chondroinductive, decellularised cartilage-derived scaffold (dcECM). The optimised vacuum assisted methodology (Vac-OS) effectively balanced the removal of immunogenic components with minimal disruption to the extracellular matrix (ECM). Therefore, the Vac-OS methodology shows excellent promise for deriving decellularised scaffolds from other dense avascular tissues, creating impact beyond skeletal regeneration Further work was carried out to optimise a methodology for the formation of 3D porous scaffolds using freeze-dried and milled dcECM granules embedded in a collagen type I scaffold. It was determined that the porous scaffolds support both cell infiltration and enhanced chondrogenesis. Moreover, the dcECM could further be digested to form a chondrogenic tissue culture coating. Both these key findings are novel, and it is proposed that upon publication these papers will form seminal contributions to the field. Additionally, the findings highlighted in this thesis provides the fundamental knowledge to develop cartilage mimetic constructs that can be used to further 4 investigate mechanisms of diseases in pathologies such as osteoporosis, opening opportunities for further funding, such as the foundation fellowship funding stream from Arthritis Research UK. There is currently no cartilage-derived bone graft substitute on the market, and with the bone graft and substitutes market being valued at 2.4 billion USD in 2016 and a CAGR of 5.5% by 2025, there is excellent potential for a revolutionary approach such as described in this thesis to fill this gap the market. Moreover, key players such as Medtronic PLC, Nuvasive, Inc and Stryker Corp adopt strategies that include collaboration with academia for the innovation of novel bone regeneration strategies, opening further opportunities for funding and commercialisation. 5 Abbreviations ABG - Autologous bone grafting ACI - Autologous chondrocyte implantation ANOVA - Analysis of variance BCA - Bicinchoninic acid bFGF - Basic fibroblast growth factor BMP - Bone morphogenic proteins CAM - Chorioallantoic membrane CD - Cluster of differentiation C-dcECM – Compressed dcECM cDNA - Complementary DNA ChM-I - Chondromodulin 1 CREB - Element-Binding Protein CTECs - Cartilage tissue-engineered constructs DAPI - 4′,6-diamidino-2-phenylindole DNA – Deoxyribonucleic acid DNase - Deoxyribonuclease DSC – Differential scanning calorimetry ECM - Extracellular matrix EGF - Endothelial Growth Factor EO - Endochondral ossification Erk - Extracellular Signal-Regulated Kinase ESC - embryonic stem cell 6 FAK - Focal adhesion kinase FBS - Foetal bovine serum FDA - Food and Drug Administration FGF - Fibroblastic growth factor FLIM - Fluorescence lifetime imaging microscopy GAG – Glycosaminoglycan GDF5 - Growth/Differentiation Factor 5 GF’s – Growth Factors H&E – Haematoxylin & Eosin hESCs - Human embryonic stem cells hPDSCs - Human periosteum-derived stem cells HPLC - High Performance Liquid Chromatography IFN-γ - Interferon- γ IL - Interleukin IM - Intramembranous iPSCs - Induced pluripotent stem cells ITS – Insulin transferrin selenium LSCM - Laser-scanning confocal microscopy MM – Micromass MSCs - Mesenchymal stem cells NBF – Neutral buffered formalin-saline NIH - National institute of health PCL – Polycaprolactone P-dcECM - Porous dcECM 7 PDCs - Periosteum-derived cells PDGF - Platelet-derived growth factor PGA – Polyglycolide PI3K - Phosphoinositide 3-kinase PLA - Polylactic acid PSU – Polysulfone qRT-PCR - quantitative reverse transcription–Polymerase Chain Reaction RIPA – Radioimmunoprecipitation RNase – Ribonuclease ROI - Regions of interest SDC - Sodium deoxycholate SDF1α - Stromal-derived factor 1 alpha SDS - Sodium dodecyl sulfate sGAG – Sulfated glycosaminoglycan SHG - Second harmonic generation TGFβ1 - Transforming growth factor beta-1 TNF-α - Tumour necrosis factor-α TNF-β - Tumour necrosis factor-β UV - Ultraviolet Vac - Vacuum assistance VEGF - Vascular endothelial growth factor Wnt- Wingless-related factors μCT - Microcomputed tomography 8 Contents Title…………………………………………………………………………………..1 Acknowledgements…………………………………..………………………...…..2 Abstract……………………………………………………………………………...3 Impact Statement…………………………………………………………………..4 Abbreviations……………………………………………………………………….6 Table of Contents…………………………………………………………………..9
Details
-
File Typepdf
-
Upload Time-
-
Content LanguagesEnglish
-
Upload UserAnonymous/Not logged-in
-
File Pages260 Page
-
File Size-