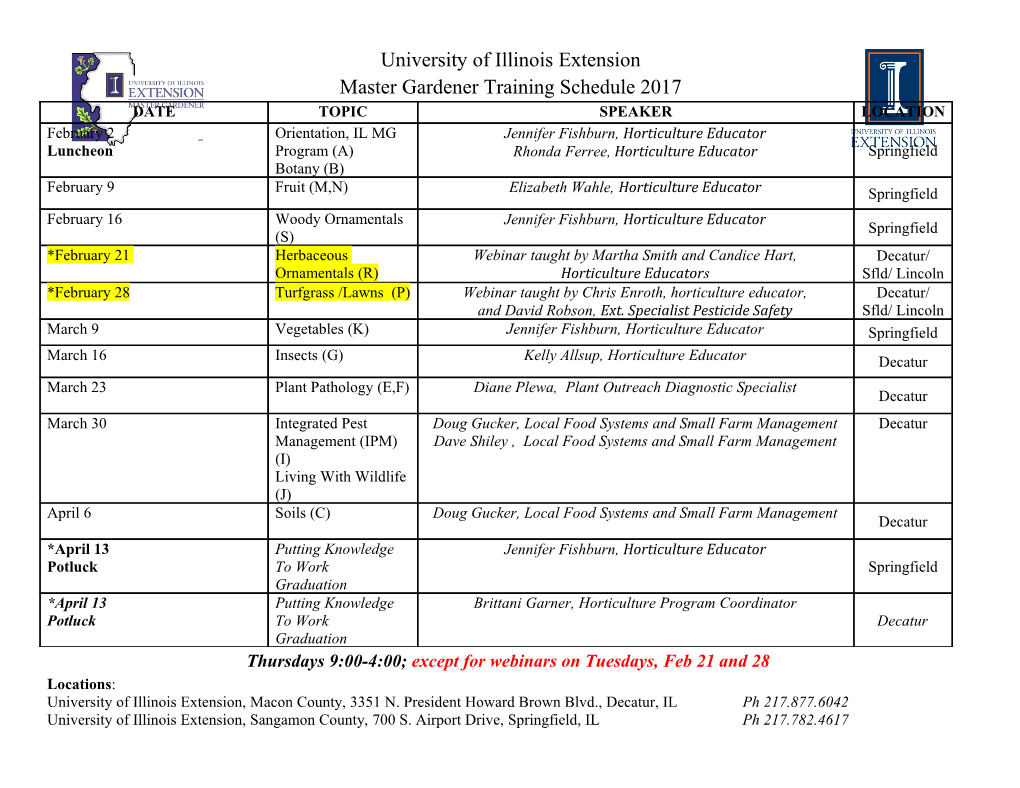
Ribosome-induced changes in elongation factor Tu SEE COMMENTARY conformation control GTP hydrolysis Elizabeth Villaa,b,1, Jayati Senguptac,1,2, Leonardo G. Trabucoa,b, Jamie LeBarronc, William T. Baxterc, Tanvir R. Shaikhc, Robert A. Grassuccid, Poul Nissene, Måns Ehrenbergf, Klaus Schultena,g, and Joachim Frankd,h,3 aBeckman Institute, University of Illinois at Urbana-Champaign, Urbana, IL 61801; bCenter for Biophysics and Computational Biology, University of Illinois at Urbana-Champaign, Urbana, IL 61801; cWadsworth Center, Empire State Plaza, Albany, NY 12201; dHoward Hughes Medical Institute, Department of Biochemistry and Molecular Biophysics, Columbia University, 650 West 168th Street, New York, NY 10032; eDepartment of Molecular Biology, University of Aarhus, Gustav Wieds vej 10C, DK-8000 Aarhus, Denmark; fDepartment of Cell and Molecular Biology, Biomedical Center, Box 596, S-75124 Uppsala, Sweden; gDepartment of Physics, University of Illinois at Urbana-Champaign, Urbana, IL 61801; and hDepartment of Biological Sciences, Columbia University, 600 Fairchild Center, Mail Code 2402, 1212 Amsterdam Avenue, New York, NY 10027 Contributed by Joachim Frank, November 12, 2008 (sent for review October 10, 2008) In translation, elongation factor Tu (EF-Tu) molecules deliver interaction with the ribosome (3, 6). So far, gate opening has only aminoacyl-tRNAs to the mRNA-programmed ribosome. The GTPase been observed in the crystallographic structure of Thermus activity of EF-Tu is triggered by ribosome-induced conformational thermophilus EF-Tu in complex with GDP and the GTPase- changes of the factor that play a pivotal role in the selection of the stimulating antibiotic methyl-kirromycin (aurodox), outside the cognate aminoacyl-tRNAs. We present a 6.7-Å cryo-electron mi- ribosome. croscopy map of the aminoacyl-tRNA⅐EF-Tu⅐GDP⅐kirromycin-bound In the present work, we focus on the ribosome-induced EF-Tu Escherichia coli ribosome, together with an atomic model of the conformational changes that trigger GTP hydrolysis on the complex obtained through molecular dynamics flexible fitting. The factor as revealed by cryo-electron microscopy (cryo-EM) stud- model reveals the conformational changes in the conserved GTPase ies. We provide structural evidence suggesting that the ribosome switch regions of EF-Tu that trigger hydrolysis of GTP, along with induces the opening of the hydrophobic gate and the reposi- key interactions, including those between the sarcin-ricin loop and tioning of His-84 of EF-Tu. Our results reconcile a large body of the P loop of EF-Tu, and between the effector loop of EF-Tu and a experimental data and explain how the ribosome controls the conserved region of the 16S rRNA. Our data suggest that GTP GTPase activity of EF-Tu in the ternary complex. hydrolysis on EF-Tu is controlled through a hydrophobic gate mechanism. Results We obtained a 6.7-Å cryo-EM map of the preaccommodated decoding ͉ GTPase ͉ flexible fitting ͉ cryo-EM ͉ ternary complex Escherichia coli 70S ribosome bound to the Phe-tRNAPhe⅐EF- Tu⅐GDP ternary complex stalled by the antibiotic kirromycin n the elongation cycle of protein synthesis, each aminoacyl- (kir) (7). We applied the recently developed molecular dynamics BIOPHYSICS ItRNA (aa-tRNA) is delivered to the mRNA-programmed flexible fitting (MDFF) method (8) to obtain an atomic model ribosome as a ternary complex with elongation factor Tu (EF- of the complex that enables the interpretation of the cryo-EM Tu) and GTP. On binding to the ribosome, aa-tRNA enters the data in unprecedented detail. The map, along with the fitted A/T state, where it occupies the A site on the 30S ribosomal atomic model of the 70S ribosome, the ternary complex, P- and subunit while still interacting with EF-Tu. When the codon– E-site tRNAs, and mRNA are shown in Fig. 1A. This map anticodon interaction is cognate, GTPase activity of EF-Tu is presents a substantial advancement in resolution over previously greatly stimulated and GTP hydrolysis occurs rapidly, inducing reported cryo-EM data (9–11), allowing us to corroborate a large conformational change of EF-Tu followed by its disso- previous observations in greater detail, and to observe new ciation from the ribosome and subsequent accommodation of contacts and changes in conformation of the complex. In par- aa-tRNA into the ribosomal A site. The irreversible GTP ticular, with the improvement in resolution, the structural fea- hydrolysis separates 2 kinetic steps of aa-tRNA incorporation, tures of EF-Tu and its interactions with the ribosome are clearly namely initial selection and proofreading, that jointly establish discernible in the density map. the overall accuracy of tRNA selection by the codon- The relative orientation between the domains of the ribo- programmed ribosome (1). Much effort has been devoted to some-bound EF-Tu with kirromycin (Fig. 1B) is most similar to elucidate the mechanism by which codon recognition in the 30S subunit leads to GTP hydrolysis by EF-Tu Ͼ75 Å away. Bio- chemical and structural studies identified some key interactions, Author contributions: M.E., K.S., and J.F. designed research; E.V., J.S., L.G.T., J.L., W.T.B., T.R.S., and R.A.G. performed research; M.E. contributed new reagents/analytic tools; E.V., but the atomic details of the mechanism have remained elusive. J.S., L.G.T., J.L., W.T.B., T.R.S., P.N., and K.S. analyzed data; and E.V., J.S., L.G.T, K.S., P.N., and In particular, the transduction of the codon recognition signal J.F. wrote the paper. and subsequent ribosome-induced EF-Tu conformational The authors declare no conflict of interest. changes leading to GTPase activation are not well understood. Freely available online through the PNAS open access option. GTP hydrolysis in EF-Tu takes place through an in-line, direct ␥ Data deposition. The atomic coordinates have been deposited in the Protein Data Bank, attack on the -phosphate by a water molecule (2). His-84 of www.pdb.org [PDB ID codes 3FIH (30S and factors) and 3FIK (50S)]. The cryo-EM density EF-Tu has been proposed to activate a water molecule for map has been deposited in the 3D-EM database, EMBL-European Bioinformation Institute nucleophilic attack on the ␥-phosphate by extracting one of its (code EMD-5036). protons (3, 4). This proposal agrees with studies identifying See Commentary on page 969. His-84 as the catalytic residue that stabilizes the transition state 1E.V. and J.S. contributed equally to this work. of GTP hydrolysis by hydrogen bonding to the attacking water 2Present address: Structural Biology and Bioinformatics Division, Indian Institute of Chem- molecule or the ␥-phosphate group of GTP (5). Crystal struc- ical Biology, Calcutta, 700032, India. tures of EF-Tu in the GTP form revealed a ‘‘hydrophobic gate’’ 3To whom correspondence should be addressed. E-mail: [email protected]. that appears to control access of His-84 to the GTP binding This article contains supporting information online at www.pnas.org/cgi/content/full/ pocket (3, 6). For His-84 to carry out its catalytic role and 0811370106/DCSupplemental. activate the water molecule, the gate would have to open on © 2009 by The National Academy of Sciences of the USA www.pnas.org͞cgi͞doi͞10.1073͞pnas.0811370106 PNAS ͉ January 27, 2009 ͉ vol. 106 ͉ no. 4 ͉ 1063–1068 Downloaded by guest on September 27, 2021 Fig. 1. Overview of EF-Tu structure bound to the ribosome. (A) Cryo-EM map of the 70S⅐fMet-tRNAfMet⅐Phe-tRNAPhe⅐EF-Tu⅐GDP⅐kir complex at a resolution of 6.7 Å shown in transparent surface. The atomic model obtained by molecular dynamics flexible fitting (MDFF) is shown in cartoon representation. (B) Atomic model of the ribosome-bound EF-Tu showing a domain orientation most similar to the closed, GTP-bound form. The switch regions are highlighted: switch I (Sw1) in blue, switch II (Sw2) in orange, and P-loop in green. The same coloring scheme is used in all figures. (C) Closeup of the GTPase domain of EF-Tu. The cryo-EM density map is displayed at a lower threshold to make the switch I density visible. Density thresholds are 2.6 (A), 2.2 (B), and 1.4 (C). the ‘‘closed,’’ GTP-bound form of EF-Tu (3, 6), as previously formed by residues Val-20 (P loop) and Ile-60 (switch I). The gate observed outside of the ribosome. The structure of EF- in its closed form is present in many crystal structures of GDP- and Tu⅐GDP⅐kir bound to the ribosome has been proposed to GTP-bound EF-Tu both bound and unbound to tRNA, indicating correspond to a state immediately after GTP hydrolysis, yet that gate opening occurs only in the presence of the ribosome. Fig. preceding the conformational change into the free, GDP-bound 2B shows the hydrophobic gate present in the crystal structure of structure and, hence, preceding EF-Tu release (11). Our data the free E. coli ternary complex (PDB ID code 1OB2; unpublished show that this EF-Tu structure indeed corresponds to an acti- data). The aurodox-bound structure, shown in Fig. 2D, displays an vated state for the hydrolysis of the nucleotide, as detailed below. open gate, where Ile-60 and the remainder of switch I are disor- Several conserved regions playing prominent roles in the work- dered and His-84 is repositioned toward the nucleotide (12), hinting ing cycle of GTPases, namely switch I (EF-Tu residues 40–62; E. that the increased GTPase activity induced by aurodox is achieved coli numbering is used throughout this article), switch II (80– by gate opening. One wing of the hydrophobic gate is formed by 100) and P loop (18–23), are found to undergo characteristic residue Val-20, which forms part of the P loop, a conserved element conformational changes in EF-Tu.
Details
-
File Typepdf
-
Upload Time-
-
Content LanguagesEnglish
-
Upload UserAnonymous/Not logged-in
-
File Pages6 Page
-
File Size-