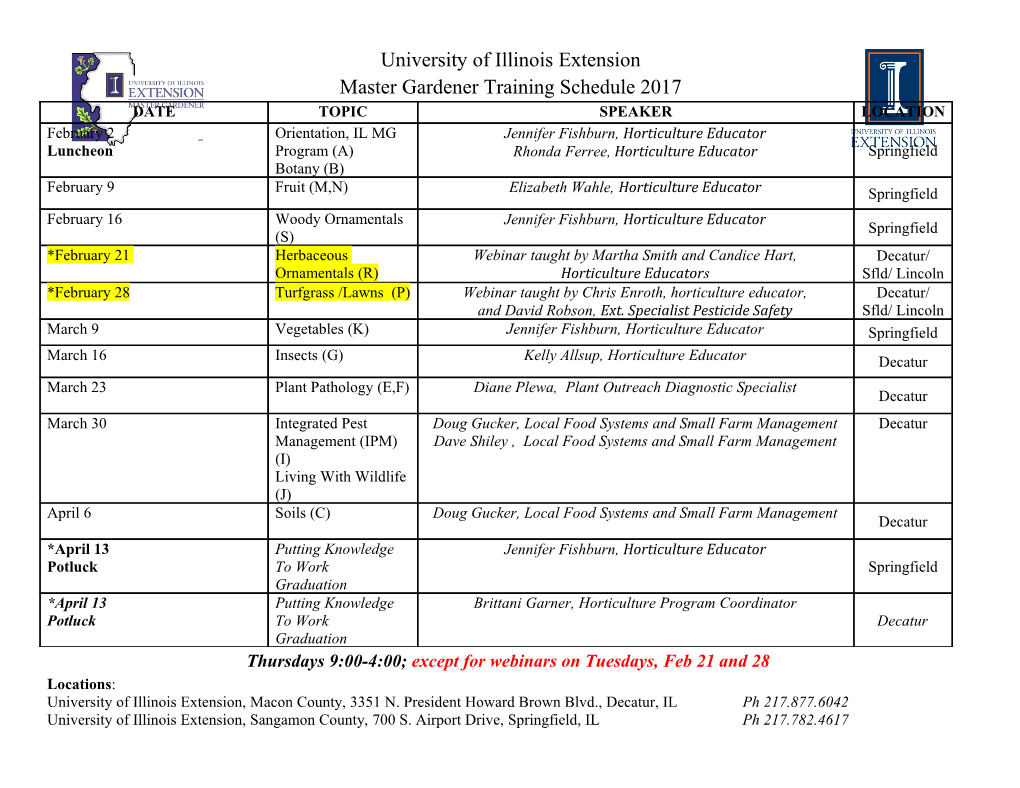
Initial sizing of a roadable personal air vehicle using design of experiments for various engine types Jaeyoung Cha and Juyeol Yun Department of Aerospace Engineering, Sejong University, Seoul, Republic of Korea, and Ho-Yon Hwang Department of Aerospace Engineering and Convergence Engineering for Intelligence Drone, Sejong University, Seoul, Republic of Korea Abstract Purpose – The purpose of this paper is to analyze and compare the performances of novel roadable personal air vehicle (PAV) concepts that meet established operational requirements with different types of engines. Design/methodology/approach – The vehicle configuration was devised considering the dimensions and operational restrictions of the roads, runways and parking lots in South Korea. A folding wing design was adopted for road operations and parking. The propulsion designs considered herein use gasoline, diesel and hybrid architectures for longer-range missions. The sizing point of the roadable PAV that minimizes the wing area was selected, and the rate of climb, ground roll distance, cruise speed and service ceiling requirements were met. For various engine types and mission profiles, the performances of differently sized PAVs were compared with respect to the MTOW, wing area, wing span, thrust-to-weight ratio, wing loading, power-to-weight ratio, brake horsepower and fuel efficiency. Findings – Unlike automobiles, the weight penalty of the hybrid system because of the additional electrical components reduced the fuel efficiency considerably. When the four engine types were compared, matching the total engine system weight, the internal combustion (IC) engine PAVs had better fuel efficiency rates than the hybrid powered PAVs. Finally, a gasoline-powered PAV configuration was selected as the final design because it had the lowest MTOW, despite its slightly worse fuel efficiency compared to that of the diesel-powered engine. Research limitations/implications – Although an electric aircraft powered only by batteries most capitalizes on the operating cost, noise and emissions benefits of electric propulsion, it also is most hampered by range limitations. Air traffic integration or any safety, and noise issues were not accounted in this study. Practical implications – Aircraft sizing is a critical aspect of a system-level study because it is a prerequisite for most design and analysis activities, including those related to the internal layout as well as cost and system effectiveness analyses. The results of this study can be implemented to design a PAV. Social implications – This study can contribute to the establishment of innovative PAV concepts that can alleviate today’s transportation problems. Originality/value – This study compared the sizing results of PAVs with hybrid engines with those having IC engines. Keywords Aircraft design, Constraint analysis, Initial sizing, Mission profile, Personal air vehicle, Roadable PAV Paper type Research paper a Nomenclature ZL = zero lift angle of attack; a fi CL = maximum lift coef cient angle of attack; Symbols max aC = cruise angle of attack; ’ fi estraight wing = Oswald s span ef ciency of straight wing; CD = drag coefficient at a zero angle of attack; ’ fi 0 eswept wing = Oswald s span ef ciency of swept wing; D = diameter of the propeller; AR = aspect ratio; PBHP = brake horsepower; ^ LE = leading edge sweep angle; RPM = revolutions per minute of the propeller; CLa = lift curve slope; b = Mach number parameter; k = ratio of the two-dimensional lift curve slope to 2p; © Jaeyoung Cha, Juyeol Yun and Ho-Yon Hwang. Published by Emerald Publishing Limited. This article is published under the Creative Commons ^c/2 = sweepback of the mid chord; fi Attribution (CC BY 4.0) licence. Anyone may reproduce, distribute, CL0 = lift coef cient at a zero angle of attack; translate andcreate derivative works of this article (for both commercial and non-commercial purposes), subject to full attribution to the original publication and authors. The full terms of this licence may be seen at The current issue and full text archive of this journal is available on Emerald http://creativecommons.org/licences/by/4.0/legalcode Insight at: https://www.emerald.com/insight/1748-8842.htm This work is supported by the Korea Agency for Infrastructure Technology Advancement (KAIA) grant funded by the Ministry of Land, Infrastructure and Transport (Grant 21CTAP-C157731-02). Aircraft Engineering and Aerospace Technology 93/11 (2021) 1–14 Received 28 April 2020 Emerald Publishing Limited [ISSN 1748-8842] Revised 21 January 2021 [DOI 10.1108/AEAT-04-2020-0073] Accepted 11 February 2021 1 Roadable personal air vehicle Aircraft Engineering and Aerospace Technology Jaeyoung Cha, Juyeol Yun and Ho-Yon Hwang Volume 93 · Number 11 · 2021 · 1–14 VTAS = airspeed; V = airspeed; PD = pitch angle of the propeller; VV = vertical speed; Vinduced = induced velocity; CD min = minimum drag coefficient; Ƞideal = ideal propeller efficiency; k = lift induced drag constant; Ƞnew = new propeller efficiency; Vstall = stall speed; Vinduced = induced velocity; CLmax = maximum lift coefficient; and WW = predicted weight of the wing; Wi = weight after the iterative process. SW = trapezoidal wing area; WFW = weight of fuel in the wing; Definitions, acronyms and abbreviations ^ c/4 = wing sweep at 25% of the mean geometric chord; HALE = high altitude long endurance; l = wing taper ratio; UAV = unmanned aerial vehicle; nz = ultimate load factor; SADF = solar aircraft design framework; W0 = design gross weight; GA = general aviation; WHT = predicted weight of horizontal tail wing; VTOL = vertical take-off landing; SHT = trapezoidal horizontal tail wing area; eVTOL = electronic vertical take-off landing; ^ HT = horizontal tail wing sweep at 25% mean UAM = urban air mobility; geometric chord; DOD = department of defense; l HT = horizontal tail wing taper ratio; MTOW = maximum take-off weight; WF = predicted weight of the fuselage; T/W = thrust to weight ratio; SFUS = fuselage wetted area; W/S = wing loading; lFS = length of the fuselage structure; P/W = power to weight ratio; dFS = depth of the fuselage structure; UIUC = university of Illinois urbana-champaign; VP = volume of the pressurized cabin section; DOE = design of experiment; D P = cabin pressure differential; LHS = Latin hypercube sampling; WMLG = predicted weight of the main landing gear; VBA = visual basic for applications; and nl = ultimate landing load factor; IC = internal combustion. Wl = design landing weight; Lm = length of the main landing gear strut; WNLG = predicted weight of the nose landing gear; Introduction nl = ultimate landing load factor; The concept of door-to-door air mobility has long been a Wl = design landing weight; dream in the aerospace industry. As a potential means of Ln = length of the nose landing gear strut; providing the freedom of rapid door-to-door mobility beyond WEI = predicted weight of the installed engine; what is widely available today via automobiles, numerous WENG = uninstalled engine weight; roadable aircraft or flying-car concepts have been proposed, N = number of engines; ENG patented, built and flown during the past half century by W = predicted weight of the fuel system; FS inventors, entrepreneurs and military and civil research Q = total fuel quantity; tot institutes (Lim et al.,2015). Q = fuel quantity in integral fuel tanks; int However, personal air vehicles (PAVs) for transportation N = number of fuel tanks; TANK have not been developed in any significant way. A new style WCTRL = predicted weight of the flight control system; b = wing span; of transportation is essential to facilitate the movement of individuals from place to place without numerous WAV = predicted weight of the avionics installation system; complications. Furthermore, the current infrastructure must be considered such that a solution that can be WUAV = predicted weight of the uninstalled avionics; seamlessly integrated into existing facilities and WEL = predicted weight of the electrical system; WFURN = predicted weight of the furnishing; infrastructure, such as highways, roads, parking lots and ’ WO = maximum take-off weight; airports, can be devised. The world s major cities are fold becoming increasingly overpopulated, and the land space mw = total weight of a folding wing; available for ground transportation is becoming extremely mnonfold = weight of the non-folding wing; w limited. Therefore, air transportation may become more ins mfold = weight of any inserted structure; important in the future (Coronado et al.,2007). mech mfold = folding mechanism weight; Based on current requirements, the scientific community mech mpin = pin weight; started to focus on general aviation (GA) and personal CLTO = lift coefficient during the take-off run; transport aircraft. NASA has recognized that personal air travel CDTO = drag coefficient during the take-off run; using an on-demand, highly distributed air transportation q = dynamic pressure; system would allow for improved mobility in congested areas in SG = ground run; the USA. Therefore, they began research on this topic through VLOF = lift off speed; the Small Aircraft Transportation System initiative (Viken m = ground friction constant; et al., 2006), focusing on airspace-related issues considering the g = acceleration due to gravity; operation of PAVs. NASA has been investigating specific 2 Roadable personal air vehicle Aircraft Engineering and Aerospace Technology Jaeyoung Cha, Juyeol Yun and Ho-Yon Hwang Volume 93 · Number 11 · 2021 · 1–14 vehicle technologies in the PAV sector
Details
-
File Typepdf
-
Upload Time-
-
Content LanguagesEnglish
-
Upload UserAnonymous/Not logged-in
-
File Pages14 Page
-
File Size-