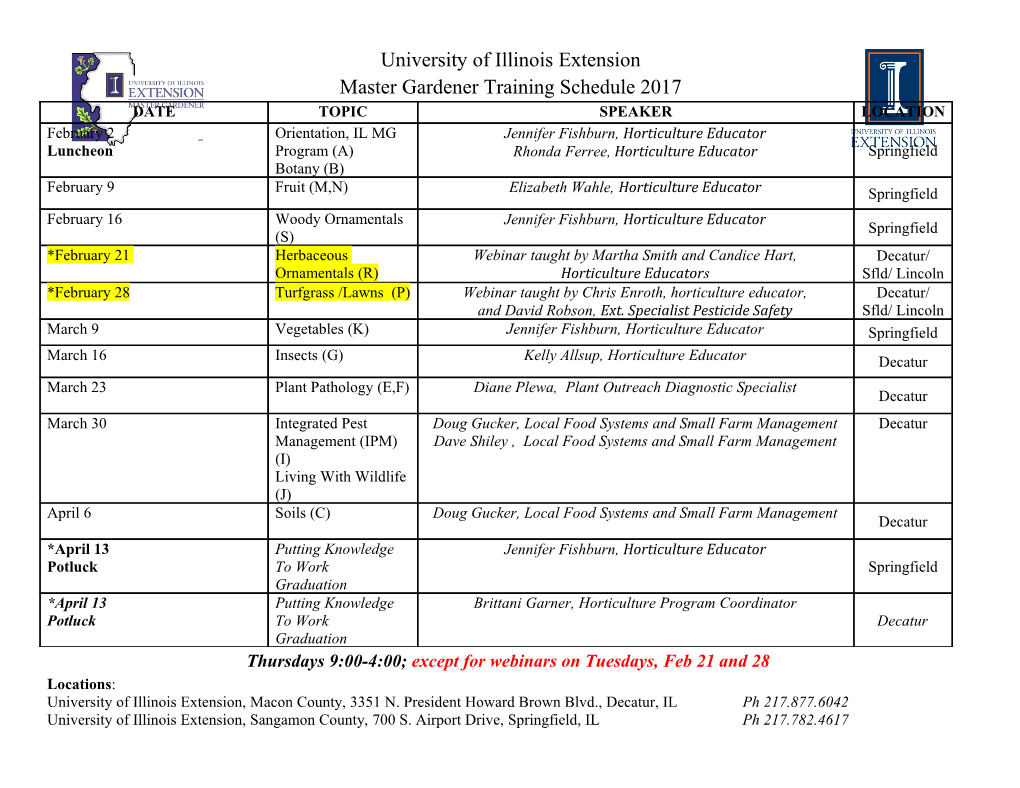
Pluto System After New Horizons 2019 (LPI Contrib. No. 2133) 7051.pdf PLUTO’S SURFACE COMPOSITION. W.M. Grundy1, D.P. Cruikshank2, S. Protopapa3, and B. Schmitt4. 1Lowell Observatory (Flagstaff AZ; w [email protected] ); 2NASA Ames Research Center (Mountain View CA); 3Southwest Research Institute (Boulder CO); 4Université Grenoble Alpes, CNRS, IPAG (Grenoble France). New Horizons’ instruments revealed spectacular Piri Planitia, a region where CH4-rich scarps appear to compositional contrasts across Pluto’s surface [1]. have retreated, exposing substrate material [21]. Fi- Some of the most striking landscapes involve the nally, H2O appears associated with a variety of pits in volatile molecules methane (CH4), nitrogen (N2), and eastern Tombaugh and further north, notably in Supay carbon monoxide (CO), frozen solid at Pluto’s low Facula. This class of H2O deposits may be indicative temperatures in the 35 to 60 K range [2]. These of eruptive processes dredging it up from the interior volatile ices have appreciable vapor pressure even at and exposing it at the surface. Additional potential such low temperatures. Their volatility supports eruptive provinces have been identified in Wright and Pluto’s complex atmosphere [e.g., 3] and it enables Piccard Mons [8,22], and in Virgil Fossae [23]. These them to sublimate and condense in response to daily regions will be discussed more in other talks. It is as- and seasonally varying patterns of insolation [4,5]. yet unclear what drives the eruptive activity, and The mobility of Pluto’s volatile ices enables significant whether it involves molten H2O or Pluto’s more transport over seasonal and longer timescales, creating volatile materials that require much less energy to mo- a diverse array of landforms ranging from the peni- bilize. Potential anti-freezes such as NH3 and CH3OH tente-like bladed terrain of Tartarus Dorsae [6,7], to have been identified spectroscopically [24,25], and the valley glaciers of eastern Tombaugh Regio may assist in the mobilization of H2O, now or earlier in [8,9,10], to the mantled, fretted, and pitted terrains at Pluto’s history. high northern latitudes [11]. Differences in the volatil- A third important class of materials on Pluto is ities of N2, CO, and CH4 ices result in very distinct re- complex organics, generally referred to as tholins. At gional distributions, with the less-volatile CH4 tending the time of the New Horizons flyby, their production to occur at high altitudes and high northern latitudes at appeared to be dominated by UV photolytic chemistry the time of the encounter, while the more volatile CO in Pluto’s upper atmosphere [e.g., 26,27,28,29]. Pho- and N2 were seen at mid-northern latitudes and in topo- tochemical products agglomerate into haze particles graphic lows [2]. A distillation sequence has been that settle out of Pluto’s atmosphere, accumulating at mapped in some regions where, initially, all three the surface. They are presumed to account for the dark volatiles condense together, but the more volatile N2 red coloration of Pluto’s equatorial maculae, but where gradually sublimates away, followed by the CO, leav- they settle on regions dominated by volatile ices they ing a CH4-rich residue [12]. One of Pluto’s primary are evidently rapidly buried by seasonal volatile trans- reservoirs of N2 ice is Sputnik Planitia [13,14], a par- port cycles. They may also interact chemically with tially-filled basin in which the N2 ice deposit is so thick molecules on Pluto’s surface, leading to further com- that it undergoes solid-state convective overturn, re- positional evolution [30]. Some forms of energetic ra- freshing its surface in a way not seen in terrestrial diation are able to penetrate through Pluto’s atmos- glaciers [15,16]. At smaller scales, the surface of phere, driving chemical evolution of the surface ices Sputnik is modified in some regions by the formation themselves, and it is possible that during certain of sublimation pits [14,17], while other regions appear epochs, this mechanism could become a dominant to be resurfaced by wind-blown dunes of CH4 ice [18]. driver of chemical evolution. A third potential source Underlying Pluto’s volatile ices is a comparatively of organics is the subsurface, where molten H2O and non-volatile substrate dominated by H2O ice. H2O ice NH3, can interact chemically with tholins incorporated is detected spectroscopically in a variety of settings, into Pluto from the protoplanetary nebula [31]. often accompanied by dark reddish material. These in- Warmer subsurface temperatures enable chemistry to clude the rugged mountains in western Sputnik Plani- proceed much faster and are likely to lead to produc- tia, that may consist of fragments of crustal material tion of biologically interesting molecules, which could buoyantly supported in Sputnik’s N2 ice. H2O ice is then be delivered to the surface environment via the also seen in Cthulhu and Krun, two examples from an various eruptive mechanisms mentioned earlier. equatorial belt of dark red maculae that appear to be Acknowledgments too warm to condense much volatile ice [19,20]. H2O ice also appears in association with dark, red deposits This work was supported by NASA’s New Hori- north of Cthulhu, such in the floors of craters and in zons project. We are grateful to the thousands of peo- ple whose work over the course of nearly two decades Pluto System After New Horizons 2019 (LPI Contrib. No. 2133) 7051.pdf made the mission a success. Names of Pluto surface 19. Earle et al. 2018. Albedo matters: Understanding features mentioned in this abstract include a mix of of- runaway albedo variations on Pluto. Icarus 303, 1- ficial and informal names. 9. 20. Binzel et al. 2017. Climate zones on Pluto and References Charon. Icarus 287, 30-36. 1. Stern et al. 2015. The Pluto system: Initial results 21. Moore et al. 2017. Sublimation as a landform- from its exploration by New Horizons. Science shaping process on Pluto. Icarus 287, 320-333. 350, 292. 22. Singer et al. 2017. Cryovolcanic resurfacing on 2. Grundy et al. 2016. Surface compositions across Pluto. American Geophysical Union, Fall Meeting Pluto and Charon. Science 351, 1283. abstract #P13F-05. 3. Gladstone et al. 2016. The atmosphere of Pluto as 23. Cruikshank et al. 2019a. Recent cryovolcanism in observed by New Horizons. Science 351, 1280. Virgil Fossae on Pluto. Icarus (in press). 4. Bertrand et al. 2018. The nitrogen cycles on Pluto 24. Cook et al. 2019. The distribution of H2O, CH3OH, over seasonal and astronomical timescales. Icarus and hydrocarbon-ices on Pluto: Analysis of New 309, 277-296. Horizons spectral images. Icarus (in press). 5. Bertrand et al. 2019. The methane cycles on Pluto 25. Dalle Ore et al. 2019. Detection of ammonia on over seasonal and astronomical timescales. Icarus Pluto’s surface in a region of geologically recent 329, 148-165. tectonism. Science Adv. (in press). 6. Moore et al. 2018. Bladed terrain on Pluto: Possi- 26. Cheng et al. 2017. Haze in Pluto’s atmosphere. ble origins and evolution. Icarus 300, 129-144. Icarus 290, 112-133. 7. Moores et al. 2017. Penitentes as the origin of the 27. Gao et al. 2017. Constraints on the microphysics bladed terrain of Tartarus Dorsa on Pluto. Nature of Pluto’s photochemical haze from New Horizons 541, 188-190. observations. Icarus 287, 116-123. 8. Moore et al. 2016. The geology of Pluto and 28. Wong et al. 2017. The photochemistry of Pluto’s Charon through the eyes of New Horizons. Sci- atmosphere as illuminated by New Horizons. ence 351, 1284-1293. Icarus 287, 110-115. 9. Umurhan et al. 2017. Modeling glacial flow on 29. Young et al. 2018. Structure and composition of and onto Pluto’s Sputnik Planitia. Icarus 287, 301- Pluto’s atmosphere from the New Horizons solar 319. ultraviolet occultation. Icarus 300, 174-199. 10. Howard et al. 2017a. Present and past glaciation 30. Grundy et al. 2018. Pluto’s haze as a surface mate- on Pluto. Icarus 287, 287-300. rial. Icarus 314, 232-245. 11. Howard et al. 2017b. Pluto: Pits and mantles on 31. Cruikshank et al. 2019b. Prebiotic chemistry of uplands north and east of Sputnik Planitia. Icarus Pluto. Astrobiology 19, (in press). 293, 218-230. 12. Schmitt et al. 2017. Physical state and distribution of materials at the surface of Pluto from New Hori- zons LEISA imaging spectrometer. Icarus 287, 229-260. 13. Protopapa et al. 2017. Pluto’s global surface com- position through pixel-by-pixel Hapke modeling of New Horizons Ralph/LEISA data. Icarus 287, 218-228. 14. White et al 2017. Geological mapping of Sputnik Planitia on Pluto. Icarus 287, 261-286. 15. McKinnon et al. 2016. Convection in a volatile ni- trogen-ice-rich layer drives Pluto's geological vigour. Nature 534, 82-85. 16. Trowbridge et al. 2016. Vigorous convection as the explanation for Pluto’s polygonal terrain. Na- ture 534, 79-81. 17. Buhler & Ingersoll 2018. Sublimation pit distribu- tion indicates convection cell surface velocities of ~10 cm per year in Sputnik Planitia, Pluto. Icarus 300, 327-340. 18. Telfer et al. 2018. Dunes on Pluto. Science 360, 992-997..
Details
-
File Typepdf
-
Upload Time-
-
Content LanguagesEnglish
-
Upload UserAnonymous/Not logged-in
-
File Pages2 Page
-
File Size-