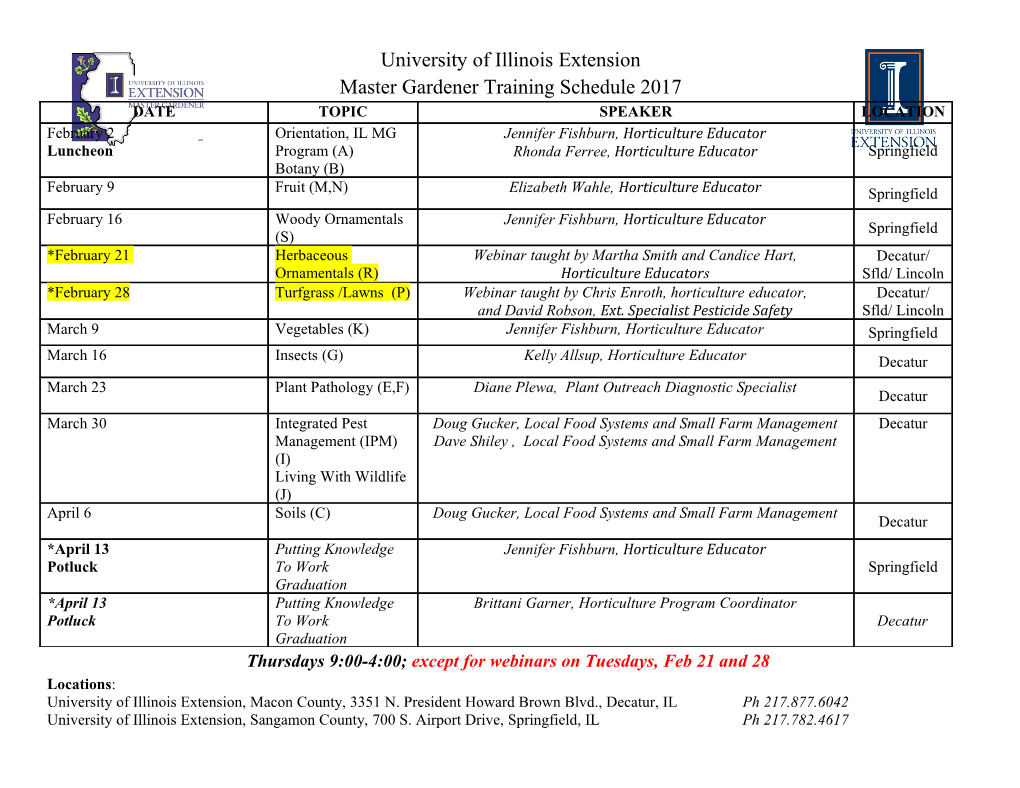
Design and Development of an External Fast Neutron Beam Facility at the Ohio State University Research Reactor Thesis By Andrew M. Zapp Graduate Program in Nuclear Engineering The Ohio State University 2019 Master‟s Examination Committee: Dr. Vaibhav Sinha, Advisor Dr. Lei Cao Copyright by Andrew M. Zapp 2019 Abstract The ability of the Ohio State University Research Reactor, OSURR, to conduct experiments from the generation of the neutron flux is important in conducting research by the University and external entities that require a flux of this magnitude. In particular, research involving a fast neutron flux is of interest due to the different interactions fast neutrons have as opposed to thermal neutrons. The OSURR is able to operate up to 500 kW, which creates a neutron flux in the order of 1013 n/cm2-s. Currently, Beam Port 2 provides a thermal neutron beam profile of 30 mm in diameter for experimentation such as neutron depth profiling, activation analysis, and evaluation of radiation damage to electronics. Beam Port 1 uses a sample area located adjacent and perpendicular to the fuel plates of the reactor for in-core irradiation. During experimentation, the remainder of Beam Port 1 must be plugged with removable concrete shielding to prevent radiation exposure that can be upwards of 1x104 rem/hr. The upgrade to Beam Port 1 consists of a collimator to shape the neutron flux from the reactor into a beam of fast neutrons, similar in diameter to Beam Port 2, in order to irradiate samples external to the reactor. In addition, mobile external shielding is designed to prevent exceeding the exposure limits of 5 rem/yr when the facility is in use. With this upgrade the research reactor has the ability to conduct simultaneous experiments with a fast and thermal neutron beam, external to the biological shielding, without releasing any harmful exposure. i Acknowledgements I would first like to thank my Nuclear Engineering graduate advisor, Dr. Vaibhav Sinha, for his help throughout this process. I would also like to thank the efforts conducted by my fellow graduate students Ibrahim Oksuz and Matthew Van Zile, who are in the Ohio State University Nuclear Engineering program, under the supervision of Dr. Lei Cao. This would not be possible without the substantial support and guidance from the staff at the Ohio State University Nuclear Reactor Laboratory. In particular, Mr. Andrew Kauffman, the Associate Director, and Mr. Joel Hatch, the Research Engineer, provided a significant amount of their time and effort in collaboration of this design. This work is supported by The Ohio State University and the Department of Energy through NEUP General Scientific Infrastructure Support Program for grant no. RU-17-13347, “A Request for Upgrade of the Ohio State University Research Reactor Beam Ports Infrastructure”. ii Vita 2005 B.A. International Studies, Russian Minor, Miami University (OH) 2008 Naval Nuclear Training Command Graduate 2007-2015 Nuclear trained Machinist Mate, USS Seawolf 2018 Presentation at SORMA XVII, “Design and Development of an External Fast Neutron Beam Facility at the Ohio State University Research Reactor”, University of Michigan Fields of Study Major Field: Nuclear Engineering iii Table of Contents Abstract i Acknowledgements ii Vita iii Fields of Study iii List of Figures vii List of Tables x 1 Introduction 1 2 Theory 4 2.1 Reactor/Source 4 2.1.1 Neutron Interactions/Properties 6 2.1.2 Gamma Interactions/Properties 8 2.2 Shielding 9 2.2.1 Neutron Shielding 9 2.2.2 Gamma Shielding 10 2.3 Collimation 12 2.3.1 Illuminator 16 2.3.2 Beam Filter 17 2.3.3 Aperture 19 3 Materials 20 3.1 Collimator Materials 20 3.1.1 Graphite Illuminator 20 3.1.2 Bismuth and Sapphire Filters 25 3.1.3 Aperture Pieces 34 3.1.3.1 Borated Cement 36 3.1.3.2 Metamic® (Borated Aluminum) 38 3.1.3.3 Lead 40 3.1.4 Aluminum 6061 as Structural Material 42 3.2 Beam Stop Shielding Materials 45 iv 3.2.1 Borated Polyethylene 45 3.2.2 Metamic® 46 3.2.3 Lead 47 4 Design 49 4.1 Existing Structure 51 4.1.1 Beam Port 2 Facility 51 4.1.2 Beam Port 1 Current Lay-out 53 4.2 MCNP Simulations 60 4.2.1 Collimator MCNP Simulations 61 4.2.2 Beam Stop MCNP Simulations 62 4.3 Design Calculations 63 4.3.1 Collimator Calculations 63 4.3.2 Shielding Calculations 67 4.3.2.1 Fast Neutrons 67 4.3.2.2 Gammas 70 4.4 Collimator Final Designs 70 4.4.1 Design Process 70 4.4.2 Beam Port 1 72 4.4.2.1 Inner Collimator 72 4.4.2.2 Outer Collimator 74 4.4.3 Beam Port 2 75 4.4.3.1 Inner Collimator 75 4.4.4 Aluminum Structural Considerations 77 4.5 External Shielding Design 80 4.5.1 Beam Stop 81 4.5.2 Rail/Lift System 84 4.5.2.1 PBC Linear Components 85 4.5.2.2 Joyce-Dayton Components 87 4.5.2.3 Fabricated Components 90 4.6 Future Work 91 5 Conclusions 93 v References 95 Appendix A: Drawings Index 99 Appendix B: MATLAB Code for Table 4-6 103 Appendix C: MCNP Code for Figure 4-9 104 Appendix D: Additional Weight and Cost Estimates 110 vi List of Figures Figure 2-1: Elastic neutron scattering collision 6 Figure 2-2: Different gamma interactions with respect to the materials Z number and gamma energy (Knoll 2010) 9 Figure 2-3: Attenuation coefficients based on energy for NaI (Knoll 2010) 12 Figure 2-4: General image plane for a source showing the image plane and distance (ASTM E803 1996) 14 Figure 2-5: Final Collimator design for the Beam Port 2 upgrade in 2012 (Turkoglu 2012) 15 Figure 2-6: Neutron Cross Sections for Carbon (Lamarsh 2001) 17 Figure 3-1: Graphite, Beam Port 1 23 Figure 3-2: Graphite, Beam Port 2 24 Figure 3-3: 3-D image of Graphite with aluminum case for Beam Port 2 25 Figure 3-4: Three single-crystal Sapphires (left) used in the 2012 upgrade to Beam Port 2 (Turklogu 2012) 26 Figure 3-5: Gamma attenuation for different thicknesses of Bismuth 27 Figure 3-6: Bismuth total cross-section for 1 - 600 meV neutron energy ranges (Freund 1983) 28 Figure 3-7: Bismuth total cross-section for 10-3 - 10 eV neutron energy ranges (Adib 2003) 29 Figure 3-8: Total neutron cross section for 209Bi, from .0125 eV – 5 MeV (NNDC) 30 Figure 3-9: Poly-crystal Bismuth molded into an aluminum holder (Turklogu 2012) 31 Figure 3-10: 3-D image of new Sapphire holder 32 Figure 3-11: Bismuth encased in Aluminum shell 33 3 6 10 Figure 3-12: σabs of He, Li, and B for a large range of neutron energies (Knoll 2010) 35 Figure 3-13: Borated Cement piece for Inner Collimator 38 Figure 3-14: Metamic® Apertures 40 Figure 3-15: Attenuation coefficients for Lead (Lamarsh 2001) 41 vii Figure 3-16: Lead Apertures 41 Figure 3-17: Stress-Strain Curves (Oberg 2012) 42 Figure 3-18: Example of Borated Polyethylene sheet drawing 46 Figure 3-19: Example of Metamic® sheet drawing 47 Figure 3-20: 3-D model of lead sheet 48 Figure 4-1: Rear View of Beam Port 2 (OSURR n.d.) 52 Figure 4-2: Overhead view of Beam Port 2 (OSURR n.d.) 53 Figure 4-3: Beam Port 1 at exit of the port (OSURR n.d.) 54 Figure 4-4: 3-D overhead view of Beam Ports inside the OSURR 55 Figure 4-5: 3-D view of Beam Ports inside the OSURR 56 Figure 4-6: 3-D cut-out of the Shutter Box Assembly in the “Open” (left) and “Shut” (right) position 57 Figure 4-7: Removal of the Shutter Box Assembly (OSURR n.d.) 57 Figure 4-8: Shutter Box Assembly when removed for maintenance (OSURR n.d.) 58 Figure 4-9: MCNP model of the collimator 60 Figure 4-10: Neutron transmittance through 4” of Bismuth from neutron energies of .0125 eV – 10 MeV 64 Figure 4-11: Neutron transmittance showing the energy range corresponding to 1/v cross section range (.0125 eV – 1 keV) 65 Figure 4-12: Final neutron flux through Bismuth 66 Figure 4-13: Collimated fast neutron flux 66 Figure 4-14: Example of drawing assembly 71 Figure 4-15: Example of individual drawing used for above assembly 72 Figure 4-16: Inner Collimator, Beam Port 1 73 Figure 4-17: Inner Collimator Aluminum Shell, Beam Port 1 73 Figure 4-18: Outer Collimator 74 Figure 4-19: Inner Collimator Aluminum Shell 75 Figure 4-20: Inner Collimator, Beam Port 2 76 Figure 4-21: Inner Collimator Aluminum Shell, Beam Port 2 76 Figure 4-22: Shield plug handling tool end (OSURR n.d.) 77 Figure 4-23: 3-D model of Aluminum shell cap design for removing the collimator 78 viii Figure 4-24: Stress and beam load case for Aluminum shell extending into the reactor pool (Oberg 2012) 79 Figure 4-25: 3-D Model of Beam Stop 82 Figure 4-26: 3-D model of Beam Stop cut-out showing Lead inserted in the back 83 Figure 4-27: Double roller pillow block data from PBC Linear (PBC Linear n.d.) 85 Figure 4-28: 3-D model of PBC linear components with bottom frame 86 Figure 4-29: 3-D model showing the removable section of railing 86 Figure 4-30: Beam Port 2 (Left) Beam Shutter without Lead components, (Right) 3-D model of Beam Shutter with Lead components (Turklogu 2012) 87 Figure 4-31: Typical machine screw jack with a lifting screw (Joyce/Dayton n.d.) 88 Figure 4-32: Machine screw jack with a traveling nut (Joyce/Dayton n.d.) 89 Figure 4-33: 3-D model of Joyce-Dayton Components 90 Figure 4-34: 3-D model of bottom frame (left) and middle frame (right) 91 Figure 4-35: 3-D Model of all components 91 ix List of Tables Table 2-1: OSURR Neutron flux data at different areas of the core (OSURR n.d.) 4 Table 2-2: Energy loss values for Hydrogen and Carbon 7 Table 3-1: Carbon nuclide data (NNDC n.d.) 21 Table 3-2: Comparison of the three filter materials and associated densities and cross sections 30 Table 3-3: Bismuth nuclide data (NNDC n.d.) 31 Table 3-4: Boron nuclide data (NNDC n.d.) 34 Table 3-5: Composition data of Borated Cement vs.
Details
-
File Typepdf
-
Upload Time-
-
Content LanguagesEnglish
-
Upload UserAnonymous/Not logged-in
-
File Pages125 Page
-
File Size-