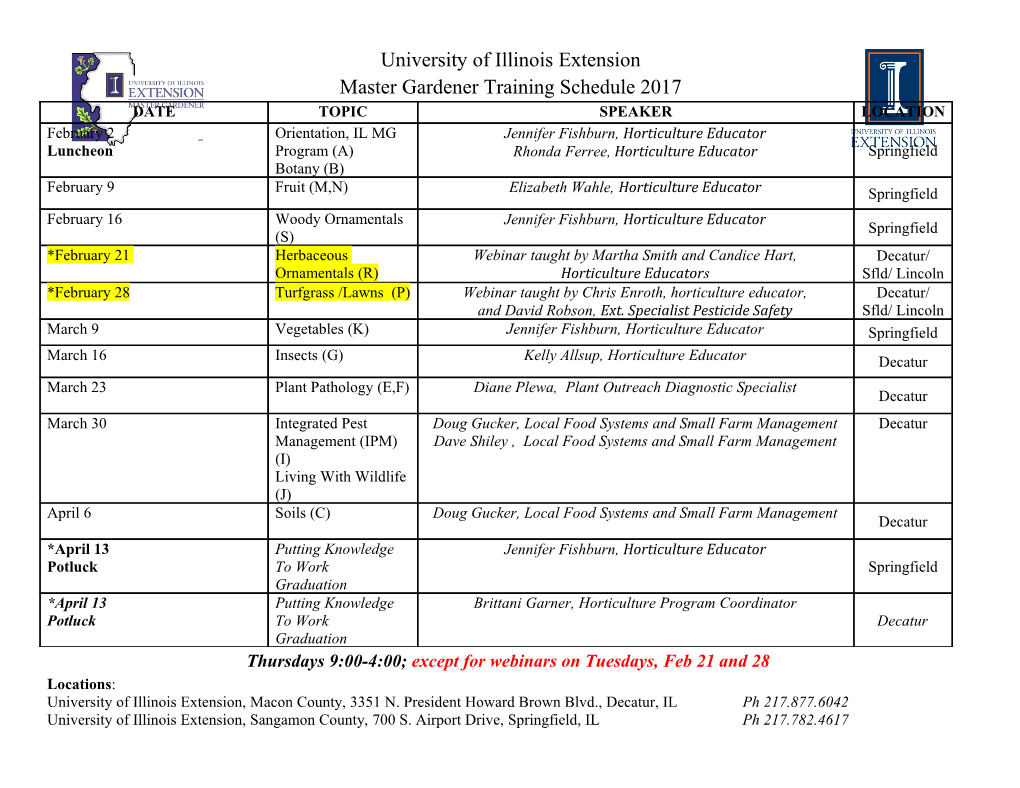
Geological Society, London, Special Publications The geomagnetic polarity timescale for the Triassic: linkage to stage boundary definitions Mark W. Hounslow and Giovanni Muttoni Geological Society, London, Special Publications 2010; v. 334; p. 61-102 doi:10.1144/SP334.4 Email alerting click here to receive free email alerts when new articles cite this service article Permission click here to seek permission to re-use all or part of this article request Subscribe click here to subscribe to Geological Society, London, Special Publications or the Lyell Collection Notes Downloaded by Biblio Geologia-Scienze Della Terra-Mi on 7 June 2010 © 2010 Geological Society of London The geomagnetic polarity timescale for the Triassic: linkage to stage boundary definitions MARK W. HOUNSLOW1* & GIOVANNI MUTTONI2 1Centre for Environmental Magnetism and Palaeomagnetism, Lancaster Environment Centre, Lancaster University, Bailrigg, Lancaster, UK LA1 4YQ 2Dipartimento di Scienze della Terra, Universita` di Milano, Via Magiagalli 34, I-20133 Milan, Italy (e-mail: [email protected]) *Corresponding author (e-mail: [email protected]) Abstract: Studies of Triassic magnetostratigraphy began in the 1960s, with focus on poorly fossilferous nonmarine red-beds. Construction of the Triassic geomagnetic polarity timescale was not consolidated until the 1990s, when access to magnetometers of sufficient sensitivity became widely available to measure specimens from marine successions. The biostratigraphically- calibrated magnetostratigraphy for the Lower Triassic is currently largely based on ammonoid zona- tions from Boreal successions. Exceptions are the Permian–Triassic and Olenekian–Anisian boundaries, which have more extensive magnetostratigraphic studies calibrated by conodont zona- tions. Extensive magnetostratigraphic studies of nonmarine Lower Triassic successions allow a vali- dation and cross-calibration of the marine-based ages into some nonmarine successions. The Middle Triassic magnetostratigraphic timescale is strongly age-constrained by conodont and ammonoid zonations from multiple Tethyan carbonate successions, the conclusions of which are supported by detailed work on several nonmarine Anisian successions. The mid Carnian is the only extensive interval in the Triassic in which biostratigraphic-based age calibration of the magnetostratigraphy is not well resolved. Problems remain with the Norian and early Rhaetian in properly constraining the magnetostratigraphic correlation between the well-validated nonmarine successions, such as the Newark Supergroup, and the marine-section-based polarity timescale. The highest time-resolution available from magnetozone correlations should be about 20–30 ka, with an average magnetozone duration of c. 240 ka, for the Lower and Middle Triassic, and about twice this for the Upper Triassic. The early pioneering work of Brunhes (1906) and Creer et al. (1954). The later authors also undertook Matuyama (1929) recognized that volcanic rocks the first published magnetostratigraphic study, recorded magnetization directions similar to the focussing on the Late Proterozoic from the UK. orientation of the present day Earth’s magnetic Palaeomagnetic work on other Triassic successions, field (i.e. of normal polarity), but also that some vol- from the USA, quickly followed (Graham 1955; canic rocks recorded older magnetization directions Runcorn 1955; Du Bois 1957), demonstrating that that were in the opposite direction (i.e. of reverse other sediments also recorded magnetizations of polarity). Motonori Matuyama was the first to both reverse and normal polarity. Radiometric suggest that these directions recorded the reversal evidence, providing convincing support that the in the main (i.e. dipole) component of the Earth’s Earth’s magnetic field polarity changes were syn- magnetic field (see discussion of early developments chronous on a global scale, was firmly established in Jacobs 1963). The first studies on the natural rema- in the early 1960s (Irving 1964; see review in nent magnetization and magnetic properties of sedi- McElhinney & McFadden 2000), which Vine & mentary rocks were conducted in the late 1930s and Matthews (1963) used in their sea-floor spreading 1940s, often with the focus on Pleistocene continen- model, linking Earth’s magnetic field polarity tal sediments (e.g. McNish & Johnson 1938; Ising changes with sea-floor magnetic anomaly lineations. 1942; Nagata 1945; Graham 1949; Torreson et al. 1949). In the 1950s, more comprehensive work on Neogene volcanic rocks showed a consistent strati- Roots of a Triassic Geomagnetic Polarity graphic pattern in the recorded polarity of magneti- Timescale (GPTS) zations, that is, a magnetostratigraphy (see Irving 1964;Hailwood1989;andMcElhinney&McFadden Most of the palaeomagnetic work in the 1950s and 2000 for a review of these early developments). 1960s was directed to providing data to support the Palaeomagnetic data from Triassic red-bed sedi- concepts of continental drift (Irving 1964). Work ments were first published by Clegg et al. (1954) and by Creer (1958, 1959) on part of the UK Triassic From:LUCAS, S. G. (ed.) The Triassic Timescale. Geological Society, London, Special Publications, 334, 61–102. DOI: 10.1144/SP334.4 0305-8719/10/$15.00 # The Geological Society of London 2010. 62 M. W. HOUNSLOW & G. MUTTONI was the earliest Triassic palaeomagnetic study that finally heralded the expansion of detailed studies placed a set of palaeomagnetic samples into strati- on the construction of a Triassic GPTS, after earlier graphic order to produce a simple magnetostrati- attempts to apply the new instrumentation to lime- graphy. stone successions of other ages (e.g. Martin 1975; The early pioneer in the development of mag- Heller 1977). The seminal magnetostratigraphic netostratigraphy for stratigraphic correlation was works of Lowrie & Alvarez (1977) and Channell A. N. Khramov, who published the seminal sum- et al. (1979) on Cretaceous limestones of the Apen- mary of on-going Russian work in 1958 (Khramov ninesand the SouthernAlps, respectively,were influ- 1958). This was primarily focused on extensive ential and were followed by work on the Triassic by studies of Neogene and Quaternary successions in Heller et al. (1988), McFadden et al. (1988) and western Turkmenistan (Cheleken Peninsula), but Steiner et al. (1989), who provided the first detailed was also significant (Irving 1964; Glen 1982) in magnetostratigraphic studies of Triassic carbonates, that it discussed fundamental magnetostratigraphic in predominantly marine successions. concepts, such as the use of multiple sections, minimum sampling requirements to define magne- tozones, and palaeomagnetic data quality. It also Early developments of the Triassic GPTS anticipated the construction of a geomagnetic The first attempts at the construction of a Triassic polarity timescale (GPTS) for dating and corre- GPTS through the 1960s and 1970s were inevitably lation. In addition, Khramov (1958) outlined a rudi- fragmentary, being based around nonmarine succes- mentary working knowledge (without details) of the sions, which were often imprecisely dated by ver- magnetostratigraphy from Upper Permian and tebrates and palynomorphs. Khramov (1963) was Lower Triassic sections in the Vyatka River region the first to attempt the construction of a Lower of the Moscow Basin. Details of this multiple- and Middle Triassic GPTS, based on the Vetluga section magnetostratigraphic study appeared sub- successions from the Moscow Basin and existing sequently (Khramov 1963), and it was quickly studies from the western literature. This was later fol- followed by studies on the Chugwater Formation lowed by attempts at a complete Triassic GPTS by in the western USA (Picard 1964) and the German McElhinney & Burek (1971),Pergamentet al. (1971), Upper Buntsandstein (Burek 1967, 1970). Pechersky & Khramov (1973), and Molostovsky The focus of Triassic magnetostratigraphic et al. (1976). studies in the 1950s to early 1980s was on terrestrial In spite of the rapid development of the GPTS for red-bed successions, since these provided natural the latest Jurassic to Pleistocene, mainly through remanent magnetizations that could be easily study of sea-floor linear magnetic anomalies, the measured on the early astatic magnetometers and absence of Triassic sea-floor largely impeded the the later fluxgate spinner magnetometers then avail- development of a detailed Triassic GPTS until the able (Collinson et al. 1957; Gough 1964). During widespread availability of SQUID magnetometers this period, the development of routine magnetic in the late 1980s. A feature that also characterizes cleaning techniques, referred to as demagnetization most of the Triassic magnetostratigraphic studies (As & Zijderveld 1958; Creer 1959; Wilson 1961) and GPTS construction prior to the 1990s is the and more rigorous analysis (i.e. using least-square common lack of true integration with detailed best fitting methods: Kirschvink 1980) of palaeo- biostratigraphies provided by the co-study of, for magnetic data, were developed into methodologies example, ammonoids and conodonts. It is the that are routinely used today. The widespread use initial expansion of such integration in the early of full demagnetization techniques, now accepted 1990s with studies such as Ogg & Steiner (1991) as standard for extracting primary magnetizations, using ammonoids, and Gallet et al. (1992, 1993) was only fully embraced in the 1970s. As such using conodonts, that the Triassic GPTS has now there is some
Details
-
File Typepdf
-
Upload Time-
-
Content LanguagesEnglish
-
Upload UserAnonymous/Not logged-in
-
File Pages43 Page
-
File Size-