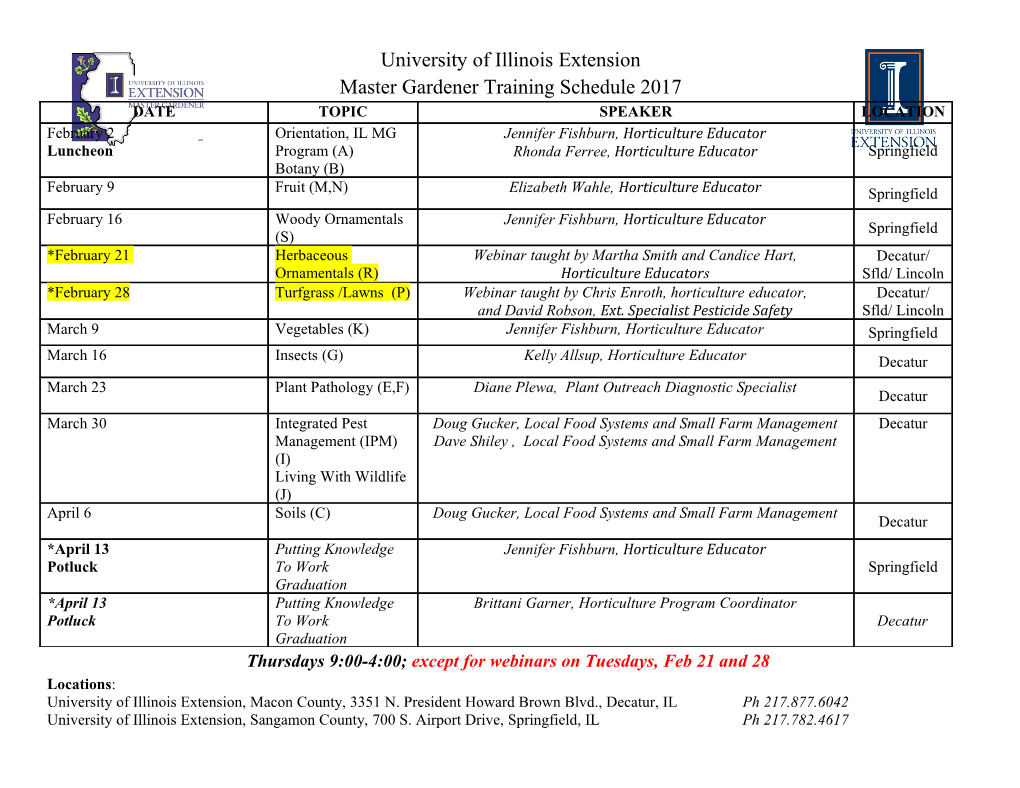
Guanine riboswitch variants from Mesoplasma florum selectively recognize 2-deoxyguanosine Jane N. Kim†, Adam Roth‡, and Ronald R. Breaker†‡§¶ †Department of Molecular, Cellular, and Developmental Biology, §Department of Molecular Biophysics and Biochemistry, and ‡Howard Hughes Medical Institute, Yale University, P.O. Box 208103, New Haven, CT 06520-8103 Edited by Jeffrey W. Roberts, Cornell University, Ithaca, NY, and approved August 21, 2007 (received for review June 22, 2007) Several mRNA aptamers have been identified in Mesoplasma florum witch aptamers for the modified nucleobase 7-aminomethyl-7- that have sequence and structural features resembling those of deazaguanine have been identified that require as few as 34 guanine and adenine riboswitches. Two features distinguish these nucleotides to form a selective and high-affinity binding pocket RNAs from established purine-sensing riboswitches. All possess short- (31). Moreover, there are three classes of aptamers for S- ened hairpin-loop sequences expected to alter tertiary contacts adenosylmethionine (SAM) (23, 24, 32) whose representatives are known to be critical for aptamer folding. The RNAs also carry nucle- more rare in bacteria than the SAM-I class of riboswitches com- otide changes in the core of each aptamer that otherwise is strictly monly found in Gram-positive bacteria (33–35). These findings conserved in guanine and adenine riboswitches. Some aptamers indicate that a far greater diversity of metabolite-sensing ribos- retain the ability to selectively bind guanine or adenine despite these witches exists that might be difficult for existing search strategies to mutations. However, one variant type exhibits selective and high- definitively identify and classify. affinity binding of 2-deoxyguanosine, which is consistent with its One possibility is that some organisms will have recently evolved occurrence in the 5 untranslated region of an operon containing riboswitch classes with aptamers that are unique in architecture or ribonucleotide reductase genes. The identification of riboswitch vari- ligand specificity. If such boutique riboswitches can easily emerge ants that bind nucleosides and reject nucleobases reveals that natural through evolution, there could be far more riboswitches than have metabolite-sensing RNA motifs can accrue mutations that expand the been discovered to date. In this report, we describe a series of diversity of ligand detection in bacteria. aptamers that are exceedingly rare among sequenced genomes and have been identified only in the bacterial species Mesoplasma allosteric RNA ͉ aptamer ͉ metabolite ͉ ribonucleotide reductase ͉ florum. One subclass of these RNAs is selective for 2Ј- transcription termination deoxyguanosine (2Ј-dG). Our findings highlight the capacity for metabolite-binding RNAs to evolve specificities toward structurally ene-control elements called riboswitches (1) are mRNA related derivatives and further demonstrate that exceptionally rare G motifs typically found in the 5Ј untranslated regions of riboswitch classes are likely to be present in some organisms. bacterial mRNAs. Riboswitches selectively bind small mole- Results and Discussion cules, and structural changes within the 5Ј untranslated regions Consensus Sequences and Structures of Guanine- and Adenine-Sens- are usually harnessed to control the expression of the adjoining ing Riboswitches. Guanine-sensing riboswitches usually reside ORF. The architectures of these RNAs are commonly formed upstream of genes involved in purine biosynthesis, salvage, and from a metabolite-binding aptamer domain and an expression transport (26). The guanine riboswitch aptamer from the Bacil- platform (2–5), although more diverse assemblies of aptamers lus subtilis xpt-pbuX mRNA exhibits a K for guanine of Ϸ5 nM. and expression platforms have been found that yield more d Ligand binding to this aptamer causes transcription termination, complex gene-control characteristics (6–9). and a similar gene-control mechanism is predicted for most Each aptamer adopts a complex secondary- and tertiary- other guanine riboswitches as well. X-ray crystallography has structured fold to form a conserved receptor for the ligand (10–17). been performed on this aptamer bound to either guanine or the This demand for precise structure formation and specific molecular functional analog hypoxanthine (10, 11). In both instances, the recognition causes the aptamer domains to be highly conserved ligands are almost completely enveloped by the RNA. Similarly, even among distantly related species. In contrast, the expression a related aptamer that binds adenine by using a similar archi- platform can adopt a variety of different structures provided it tecture also engulfs the ligand (11). maintains its responsiveness to the occupation state of the aptamer The tight ligand-binding pocket of this aptamer class is formed domain. by conserved nucleotides at the junction of three stems termed P1, Computer-aided searches based on conserved RNA sequences P2, and P3 (Fig. 1A). When the ligand is bound, the aptamer adopts and structures have been used to identify representatives of nu- a conformation with the P2 and P3 stems extending parallel to one merous riboswitch classes (18–24). However, these bioinformatics another. This structure is held in place by Watson–Crick base- algorithms can fail to identify variants of known riboswitch classes pairing interactions and other hydrogen bonds formed between the that differ substantially from the established aptamer consensus. loops of these stems, called L2 and L3. Most of the highly conserved Furthermore, there could be exceedingly rare classes of riboswitch nucleotides forming the ligand-binding core of the aptamer are aptamers or exceptionally small aptamers that will be missed by existing bioinformatics algorithms because there are too few rep- resentatives for comparison or they have too few conserved fea- Author contributions: J.N.K., A.R., and R.R.B. designed research; J.N.K. and A.R. performed tures. Given these limitations, many new riboswitch classes might research; J.N.K., A.R., and R.R.B. analyzed data; and J.N.K., A.R., and R.R.B. wrote the paper. remain undiscovered, and the true number of metabolite-sensing Conflict of interest statement: R.R.B. is a cofounder of BioRelix, a biotechnology company aptamer folds could greatly exceed the many types published to that has licensed riboswitch technology from Yale University for antibiotics development. date (25). This article is a PNAS Direct Submission. Indications that there are many variant, small, or rare riboswitch Freely available online through the PNAS open access option. classes to be discovered come from several recent reports of new Abbreviations: 2Ј-dA, 2Ј-deoxyadenosine; 2Ј-dG, 2Ј-deoxyguanosine; 2Ј-d-2,6-DAP, 2Ј- riboswitch classes. For example, a single C-to-U mutation within deoxy-2,6-diathinopurine nucleoside. the core of guanine riboswitches can change the specificity of ligand ¶To whom correspondence should be addressed. E-mail: [email protected]. binding to adenine (11, 26–30). Also, two related types of ribos- © 2007 by The National Academy of Sciences of the USA 16092–16097 ͉ PNAS ͉ October 9, 2007 ͉ vol. 104 ͉ no. 41 www.pnas.org͞cgi͞doi͞10.1073͞pnas.0705884104 Downloaded by guest on September 29, 2021 Fig. 1. Sequence and structural features of guanine A J1-2L2 J2-3 L3 J3-1 riboswitch aptamers and several newly found RNAs. (A) P1 P2P2’ P3 P3’ * P1’ Sequence alignment comparing the xpt guanine ribos- xpt (phosphoribosyltransferase) GAACACUCAUAUAAUCGCGUGGAUA-UGGCACGCAAGUUUCUACCGGGUA-CCGUAAAUGUCCGACUAUGGGUGAGCA witch aptamer sequence from the xpt-pbuX mRNA from I-A (ribonucleotide reductase) AGAAACUUAUACAG-GGUAGC-AUAAUGGGCUACU-GACCCCGCCUUCAAACCUA--UUUGGAGACUAUAAGUGAAAA I-B (unknown lipoprotein) AGAAACUUAUACAG-GGUAGC-AUAAUGGGCUACU-GACCCCGCCAUGAAACCUA--UUUCAUGACUAUAGGUCUUUA B. subtilis with related sequences from Mesoplasma flo- II-A (phosphate transporter) AAAAACUUAUACAG-GGUAGC-AUAAUGGGCUACU-GAA-CCGCCCCGGGACCAA--UCUCGGGACUAUAAGUGUGUA rum (types I, II, III, and IV) and from Oenococcus oeni, II-B (unknown lipoprotein) AAAAACUUAUACAG-GGUAGC-AUAUUGGGCUACU-GUU-CCGCCUCAAGACCAA--UCUUGAGACUAUAAGUGUAAA Vibrio sp., Vibrio splendidus, and Leuconostoc mesen- III-A (glycerol-3-P transport) AUUAACUUAUACAU-GAUAAC-AUAUCGGGUUGUC-GAC-CUGCCUUAAGACCGA--UCUUAAGACUAUAAGAAAAUA teroides (types V-A, V-B1, V-B2, and V-C, respectively). III-B (GMP synthase) AAAAACUUAUACAU-GACAAC-AUAUUGGGUUGUC-GAC-CUGCCUCUGGACCUA--UCCUUAGACUAUAAGCGU--- The known or putative functions of the genes immedi- III-C (xylulose transporter) AAAAACUUAUACAU-GACAAC-AUAACGGGUUGUC-GAC-CUGCCUUAGGACCCA--UCCAAAGACUAUAAGCGCAGA ately downstream of each sequence are noted as pre- IV-A (xanthine/uracil permease) GAAAACUUGUAUAA-UCCUUC-AUAUCGGGAAGGA-GUCUCUACCUAACA- CCAA--UGUUAG-AUUAUGAGUUUUAU dicted elsewhere (54). Nucleotides corresponding to V-A (IMP dehydrogenase) CCAAGCAGGUAUAUC-GUCGG-AUAAUGGCUGACA-GUUUCUACCCAACA- CCAA--UGUUGG-ACUAUCUGUGGAUG pairing regions P1, P2, and P3 are shaded blue, green, V-B1 (xanthine/uracil permease) UAAAUGCUGUAUAUAUCUAGUGAUA-UGGACUGGAUGUUUCUACUACCGAGCCUAC-CUUGGUGACUACAGUUUUUAG V-B2 (xanthine/uracil permease) UAAAUGCUGUAUAUAUCUAGUGGUA-UGGACUAGAUGUUUCUACUACCGAGCCUAC-CUUGGUGACUACAGUUUUUAG and orange, respectively. Nucleotides corresponding to V-C (xanthine/uracil permease) UUAGC-UGAUAUAGU-AUCGA-AUAAUGGUCGAUU-GUUUCUAGCCAGCA- CCCA--UGCUGGAACUAUCAUAAACA- loop regions (L) and joining regions (J) also are identi- fied. The asterisk
Details
-
File Typepdf
-
Upload Time-
-
Content LanguagesEnglish
-
Upload UserAnonymous/Not logged-in
-
File Pages6 Page
-
File Size-