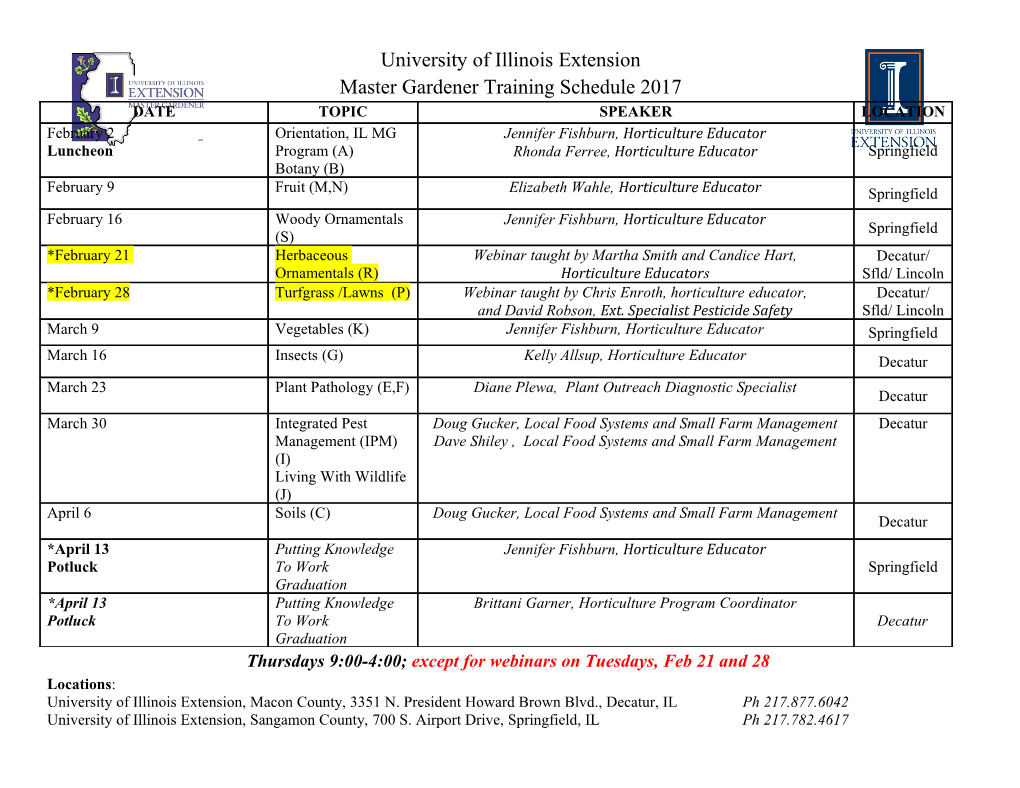
Eindhoven University of Technology MASTER Energy analysis and plant design for ethylene production from naphtha and natural gas van Gijzel, R.A. Award date: 2017 Link to publication Disclaimer This document contains a student thesis (bachelor's or master's), as authored by a student at Eindhoven University of Technology. Student theses are made available in the TU/e repository upon obtaining the required degree. The grade received is not published on the document as presented in the repository. The required complexity or quality of research of student theses may vary by program, and the required minimum study period may vary in duration. General rights Copyright and moral rights for the publications made accessible in the public portal are retained by the authors and/or other copyright owners and it is a condition of accessing publications that users recognise and abide by the legal requirements associated with these rights. • Users may download and print one copy of any publication from the public portal for the purpose of private study or research. • You may not further distribute the material or use it for any profit-making activity or commercial gain Process Engineering Multiphase Reactors group (SMR) Department of Chemical Engineering and Chemistry Den Dolech 2, 5612 AZ Eindhoven P.O. Box 513, 5600 MB Eindhoven The Netherlands www.tue.nl Graduation committee Prof. Dr. Ir. M. van Sint Annaland Dr. F. Gallucci Dr. V. Spallina (supervisor) Energy analysis and plant design for ethylene production Dr. T. Noël (external member) I. Campos Velarde from naphtha and natural gas Author R.A. van Gijzel MSc. thesis Date June 2016 Department of Chemical Engineering and Chemistry – Multiphase Reactors Group Technische Universiteit Eindhoven University of Technology ii Technische Universiteit Eindhoven University of Technology Abstract Global ethylene demand, especially in the Asia-Pacific region, is forecasted to grow with a CAGR of 3.5 % for the period from 2013 to 2019. In order to supply this increasing demand, ethylene production capacity has to be increased. Therefore existing plants have to be scaled up and or additional ethylene production plants have to be built. On the other hand further developments, such as advancements in research, strict environmental regulations, and the need for sustainable and energy efficient processes, result in the search for alternative ethylene process technologies to fill this capacity gap. At first an extensive literature study was performed on commercially available and potential ethylene production process technologies. This literature study showed that a lot of research is performed on this subject and that there are numerous options. These process technologies can be classified into fossil fuel based routes and ‘green’ renewable feedstock based routes. These green routes are thoroughly researched due to fossil fuel, mainly crude oil, depletion and the fact that the conventional ethylene production technology, naphtha steam cracking, has a high CO2 emission. From these green routes only bio-ethanol from biomass, e.g. sugarcane, to ethylene (BETE) is momentarily commercially available, profitable and capable of producing high quantities of ethylene. The highest capacity BETE plant in the world produces 200,000 t/y, which is still only 20 % of the capacity of an average naphtha steam cracker plant. Another disadvantage is the huge agricultural land requirement to grow the crops. Fossil fuel based routes deliver multiple commercially available processes, such as coal to olefins (CTO) via syngas to methanol production. From an economical point of view, together with coal abundance this is a good option. However, from an environmental point of view, this is a step back due to the higher CO2 emissions. That leaves natural gas and crude oil, of which the latter is refined into naphtha. At the moment 74 % of the ethylene produced in Europe comes from naphtha steam cracking, 71 % of the ethylene produced in the U.S. comes from natural gas via ethane steam cracking. As mentioned before, both these technology processes are very energy intensive and have high CO2 emissions. Shale gas revolution and consequently low prices of natural gas, and the large natural gas reserves result in several studies regarding the exploit of methane. One of promising process technologies, is the oxidative coupling of methane (OCM) to ethylene as an alternative. Two main obstacles have prevented OCM to be commercialized in industrial application, the first is the relatively low ethylene concentration in output gases and determining how to convert methane to C2+ with high selectivity. Despite extensive research, still many fundamental aspects about this process remain unknown. This work is part of the European project MEthane activation via integrated MEmbrane REactors (MEMERE), which is a four year research and innovation project in which eleven industrial and higher education partners work together at methane activation towards C2+. The main focus of MEMERE is the air separation through novel MIEC membranes within a reactor operated at high temperature, and hopefully achieving higher yield compared with a conventional reactor, combined with improved energy iii Technische Universiteit Eindhoven University of Technology efficiency in this multifunctional unit. Therefore this thesis assesses the energy analysis, environmental and economic performance (in terms of ethylene yield and energy efficiency) of the main conventional ethylene production process and the same for a non-membrane OCM reactor with a separate cryogenic air separation unit on site. This assessment consists of the basic design and optimization of complete, full-scale plants, built around the reactor design at different operating conditions (e.g. temperature, reactant flow and energy balance). In this thesis the following main plant configurations are modelled in Aspen Plus, each with adjustments to investigate different parameters and perform sensitivity analyses: - Model 1: The benchmark technology of the naphtha steam cracker - Model 2: The oxidative coupling of methane with air separation unit (ASU), without fuel recycle - Model 3: The oxidative coupling of methane with air separation unit, with fuel recycle iv Technische Universiteit Eindhoven University of Technology Contents Abstract iii 1 General Introduction 1 1.1 Project Motivation 1 1.4 Objectives and aim of this work 2 1.5 MEMERE 2 2 Literature review 3 2.1 Background 3 2.2 Research questions 3 2.3 State of the art ethylene production processes 5 2.4 Discussion 17 3. Energy analysis and plant design naphtha cracker 21 3.1 Theory 21 3.2 Process Flow Diagram 27 3.3 PFD section I 29 3.4 PFD section II 35 3.5 PFD section III 37 3.6 PFD section IV 39 3.7 Recycle, combustion and power generation 46 3.8 Emissions and waste 48 3.9 Coke deposition and storage 48 3.10 Energy analysis 50 3.11 Aspen Plus model 53 3.12 Steam cracking of ethane 54 4 Energy analysis and plant design for OCM 55 4.1 Introduction 55 4.2 Theory 56 v Technische Universiteit Eindhoven University of Technology 4.3 Aspen Plus model 61 4.4 ASU model 64 4.5 OCM part I 70 4.6 Energy analysis and plant design OCM part II 80 4.7 Emissions and waste 92 4.8 Sensitivity analysis recycling methane and ethane 93 4.9 Pressure sensitivity analysis 98 4.9 Temperature sensitivity analysis 104 4.10 Pinch analysis 105 4.11 Aspen Plus model 106 5 Techno-economic analysis 108 5.1 Base case economic analysis 109 5.2 Sensitivity analysis on economics 110 6 Summary and Conclusions 112 7 Recommendations 117 8 Acknowledgements 119 9 Bibliography 120 10 Appendix 124 vi Technische Universiteit Eindhoven University of Technology 1 General Introduction One of world’s largest produced raw materials in the petrochemical industry is ethylene, which is the simplest olefin (C2H4). Ethylene has various direct and indirect applications, for instance HDPE, LLDPE and LDPE or as petrochemical intermediate in order to produce PET, solvents, cosmetics, paints, packaging, etc. Also various chemical components such as ethylene dichloride, ethylene oxide and ethylbenzene are extracted from ethylene. Global ethylene capacity is estimated to rise from 167 million metric tons per year (Mtpy) in 2014 to 208.5 Mtpy in 2017, with the US and China producing one-third of the additional capacity over the forecasted coming years [1]. As the demand for ethylene is still growing, this secures ethylene production and its optimization to be an ever-interesting topic. 1.1 Project Motivation Currently, the main operation (>95 % in Western Europe) involving the production of ethylene is performed by steam cracking of larger hydrocarbons as feedstock, mainly ethane and naphtha [2]. Steam cracking introduces smaller hydrocarbons and unsaturation by the use of very high temperatures between 750-1000 °C. Reaction 1.1 below shows a simplification of ethane cracking to ethylene: CHCHH 2 6o 2 4 2 (1.1) Ethane has, besides methane, the highest endothermic heat of cracking, +4893 kJ/kg. For propane the corresponding amount is +4295 kJ/kg and for a high-molecular-mass n-alkane around 1364 kJ/kg [3]. The breaking of the bonds of the higher hydrocarbons, and thus the endothermic reactions makes the steam cracking a highly energy intensive process. Specifically, the pyrolysis section of a naphtha steam cracker consumes approximately 65% of the total process energy required for the plant [4]. According to Ren et al. (2006) the overall steam cracking process is famous for being the most energy-consuming process in chemistry because it globally uses 8% of the total primary energy use in the chemical sector. Besides cracking, other energy-intensive and costly processes such as repeated compression, cryogenic distillation and multiple water quenching steps are required to eventually produce PE grade purity. Another disadvantage of steam cracking is that the process contributes to environmental pollution by producing approximately 180-200 Mtpy of CO2 worldwide [4].
Details
-
File Typepdf
-
Upload Time-
-
Content LanguagesEnglish
-
Upload UserAnonymous/Not logged-in
-
File Pages137 Page
-
File Size-