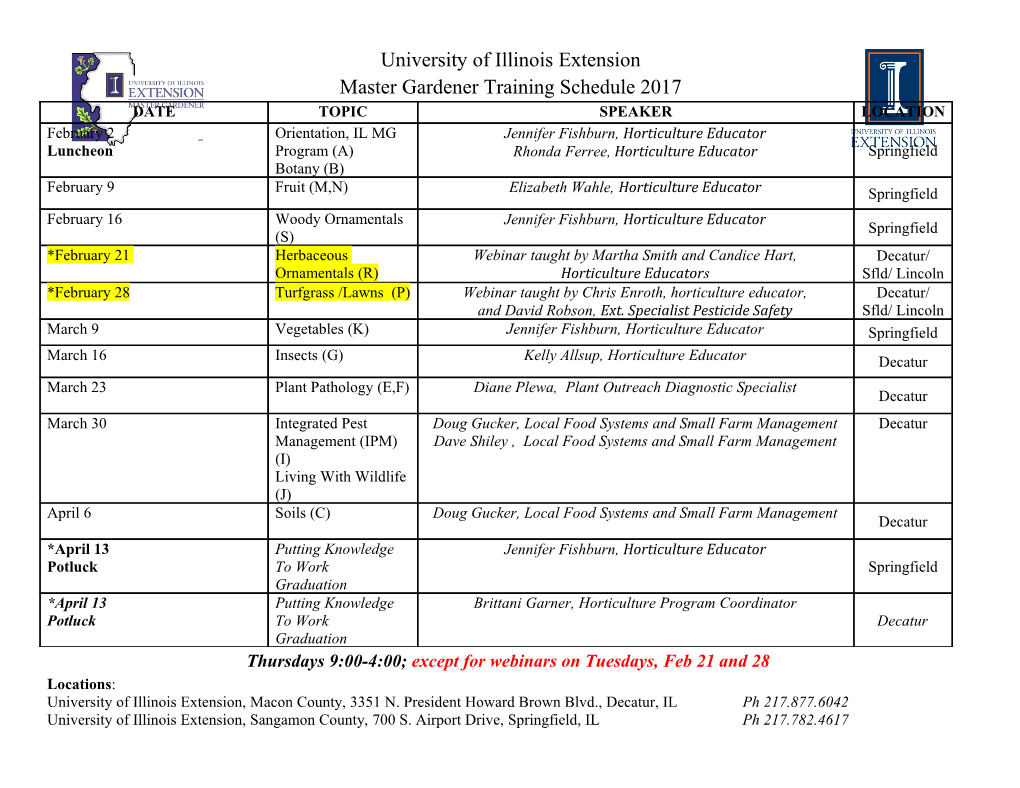
Lønningsbekk 2014 WATER QUALITY AND BIODIVERSITY OF LØNNINGSBEKK CREEK Group 6 Siri Vatsø Haugum Eihab Idris Eugene Kitsios Imelda Rantty Kåre Andreas Thorsen The University of Bergen BIO 300 UiB Group 6 : Siri Vatsø Haugum, Eihab Idris, Eugene Kitsios, Imelda Rantty, Kåre Andreas Thorsen 1 Lønningsbekk 2014 Table of Contents 1. Introduction………………………………………………………………………………….. 4 1.1 The environmental impact of concrete production……………………….. 4 1.2 Abiotic factors ………………………………………………………………………………. 5 1.3 Thermotolerant Coliform Bacteria ………………………………………………… 6 1.4 Phosphorus ………………………………………………………………………………….. 6 1.5 Benthic macroinvertebrates as ecological indicators …………………… 7 1.6 Aim of study 8 2. Materials and Methods ……………………………………………………………….. 9 2.1 Study area ……………………………………………………………………………………. 9 2.2 Sampling Procedures ……………………………………………………………………. 12 2.2.1 Thermotolerant Coliform Bacteria ………………………………………………… 12 2.2.2 Phosphorus…………………………………………………………………………………… 12 2.2.3 Temperature, conductivity, pH, dissolved oxygen, salinity and 13 velocity ………………………………………………………………………………………… 2.2.4 Creek Flow Rate …………………………………………………………………………… 13 2.2.5a Biodiversity …………………………………………………………………………………. 14 2.2.5b Laboratory investigations ……………………………………………………………. 14 2.2.5c Biodiversity analysis ……………………………………………………………………. 15 3. Results …………………………………………………………………………………………. 17 3.1 Thermotolerant Coliform Bacteria ………………………………………………… 17 3.2 Phosphorus ………………………………………………………………………………….. 18 3.3 Temperature, conductivity, pH, dissolved oxygen, salinity and 20 velocity …………………………………………………………………………………………. 3.4 Biodiversity analysis ……………………………………………………………………… 23 3.5 Physical observations……………………………………………………………………. 26 4. Discussion ……………………………………………………………………………………. 29 4.1 Abiotic factors ………………………………………………………………………………. 29 4.1.1 Conductivity …………………………………………………………………………………. 29 4.1.2 pH ………………………………………………………………………………………………… 30 4.1.3 Phosphorus ………………………………………………………………………………….. 30 4.1.4 Other factors ………………………………………………………………………………… 31 4.2 Biotic factors ………………………………………………………………………………… 32 4.2.1 Thermotolerant coliform bacteria ………………………………………………… 32 4.3 Macro-invertebrate biodiversity metrics …………………………………….. 34 4.3.1 Diversity indices ……………………………………………………………………………. 34 4.3.2 Sensitivity indices …………………………………………………………………………. 36 4.4 Interpretation of statistical analysis……………………………………………… 37 4.5 Water quality at Lønningsbekk creek …………………………………………… 39 4.6 Recommendations……………………………………………………………………….. 39 BIO 300 UiB Group 6 : Siri Vatsø Haugum, Eihab Idris, Eugene Kitsios, Imelda Rantty, Kåre Andreas Thorsen 2 Lønningsbekk 2014 5. Conclusion…………………………………………………………………………………….. 41 6. Acknowledgements ……………………………………………………………………… 42 7. References ………………………………………………………………………………….. 43 8. Appendix A …………………………………………………………………………………… 50 9. Appendix B …………………………………………………………………………………… 53 10. Appendix C …………………………………………………………………………………… 54 11. Appendix D ………………………………………………………………………………….. 55 12. Appendix E …………………………………………………………………………………… 56 13. Appendix F …………………………………………………………………………………… 57 14. Appendix G ………………………………………………………………………………….. 58 15. Appendix H ………………………………………………………………………………….. 61 16. Appendix I ……………………………………………………………………………………. 62 BIO 300 UiB Group 6 : Siri Vatsø Haugum, Eihab Idris, Eugene Kitsios, Imelda Rantty, Kåre Andreas Thorsen 3 Lønningsbekk 2014 1 Introduction Access to water is crucial for all life on earth. However, the growing human population is threatening our water resources, as demands for high quality water for domestic and economic purposes grow stronger (UNEP GEMS, 2008). Human activities, such as agricultural production, industrial production and mining, negatively affect our water quality all over the globe. Not only do these activities impact the aquatic ecosystem, but they also threaten the availability of fresh water for human consumption. Promoting sustainable use of water resources and providing safe water to people around the world are therefore key objectives of the Millennium Development Goals. Water is the basis of every healthy aquatic ecosystem. As water quality decreases, organisms are affected and ecosystem services may be lost. This impacts humans in many ways, as we depend on the supply of clean and usable water for consumption, industry and recreation (UNEP GEMS, 2008). 1.1 The environmental impact of concrete production Concrete is a construction material consisting of a mixture of cement, sand, gravel and water that hardens to rock-like consistency (Mehta and Monteiro, 2013). The production of concrete drives a huge industrial sector that is regarded as one of the largest consumers of natural resources in the world. The annual demand for concrete is 11.5 billion tons per year and is expected to grow in future (EPN, 1993). Concrete pollution at Lønningsbekk creek in Bergen (Norway) has been a problem for several years and it is the reason for the current study. Previous research on the water quality of Lønningsbekk has also been conducted in 2013 (Ghebretnsae et al., 2013), which will allow for comparisons between the data of 2013 and 2014. Concrete industry consumes a phenomenal amount of a billion tons of water each year. It is also estimated that the average water consumption at batching plants is around 500 gallons per truck. Given these numbers, it is unsurprising that concrete production contributes to water pollution in several ways; the most serious concern being the wash water from equipment cleaning which is usually discharged to setting ponds in order to settle the solids. If the wash water from batching plants finds its way into water bodies, it causes considerable damage to aquatic life. The reason is that this water is alkaline, sometimes reaching a pH of 12. Alkaline water is BIO 300 UiB Group 6 : Siri Vatsø Haugum, Eihab Idris, Eugene Kitsios, Imelda Rantty, Kåre Andreas Thorsen 4 Lønningsbekk 2014 toxic to fish, causing a wide range of adverse effects on fish gills, eyes, skin and physiology. Other forms of aquatic life are also affected (EBN, 1993; Davies et al., 2010). Concrete production causes water pollution in other ways as well, for example, the aggregate, sand and concrete dust can contribute sediments to nearby water bodies. Concrete sediments can cloud water bodies and increase the treatment cost for water supplies. Moreover, it inhibits plant growth and negatively affects the breeding sites for fish. In extreme cases, these sediments may alter the water flow, thus increasing the potential for floods. The chemical admixtures of the manufacturing process could be washed to water bodies, where they reduce the levels of oxygen available to aquatic life. Spills and leaks of fuels and lubricants can result in hydrocarbon runoff, a serious water pollutant. Finally, concrete pollution could affect human health through the consumption of polluted water and contaminated fish (EBN, 1993; Babor and Judele, 2009). 1.2 Abiotic factors The physical conditions of an aquatic ecosystem, such as temperature, conductivity, pH and dissolved oxygen (DO) directly influence the living organisms in the water body. Temperature influences the rates of biological and chemical processes. All aquatic organisms, from microbes to fish, rely on certain temperature ranges for their optimal health (EPA, 2012e). Sufficient DO is essential for growth and reproduction of aerobic aquatic organisms. The pH of aquatic systems is one of the most important factors determining the distribution of species, because even small changes in pH can cause physical stress to organisms adapted to certain levels (EPA, 2012b). The velocity of a water body can significantly affect its ability to assimilate and transport pollutants. Thus, measurement of velocity is crucial in any assessment program. It enables the prediction of movement of compounds (particularly pollutants) within water bodies, including ground waters (Chapman and Kimstach, 1996). Conductivity in water is affected by the presence of inorganic dissolved solids, as well as temperature. In streams and rivers, it is affected primarily by the geology of the area through which the water flows. The conductivity of most freshwaters ranges from 10 to 1000 µS/cm. Values exceeding 1000 BIO 300 UiB Group 6 : Siri Vatsø Haugum, Eihab Idris, Eugene Kitsios, Imelda Rantty, Kåre Andreas Thorsen 5 Lønningsbekk 2014 µS/cm are an indicator of polluted waters or water bodies that receives large quantities of run-off (EPA, 2012). 1.3 Thermotolerant Coliform Bacteria The count of Thermotolerant Coliform Bacteria (TCB) is often used as an indicator of the quality of recreational water (Statens Forurensningstilsyn, 2005). The bacteria are found in the intestines of warm blooded animals. A high number of TCB in the water can therefore indicate the presence of faecal contamination (Edberg et al., 2000). A high intake of TCB will generally be non-fatal for healthy adults, although the YOPI (Young, Old, Pregnant and Immunosuppressed) population can develop serious illness following an infection. Often, an infection with Escherichia coli (a TCB) will cause diarrhoea and stomach pain, but some strains are more pathogenic and can cause serious illness with symptoms like bloody diarrhoea and haemolytic-uremic syndrome (Minnesota Department of Health, 2009). Limitations to the amount of TCB countable colonies in drinking and bathing water have been set to avoid public health problems (Statens Forurensningstilsyn, 2005). 1.4 Phosphorus Phosphorus, together with nitrogen, is an essential element for all aquatic plant life on earth
Details
-
File Typepdf
-
Upload Time-
-
Content LanguagesEnglish
-
Upload UserAnonymous/Not logged-in
-
File Pages64 Page
-
File Size-