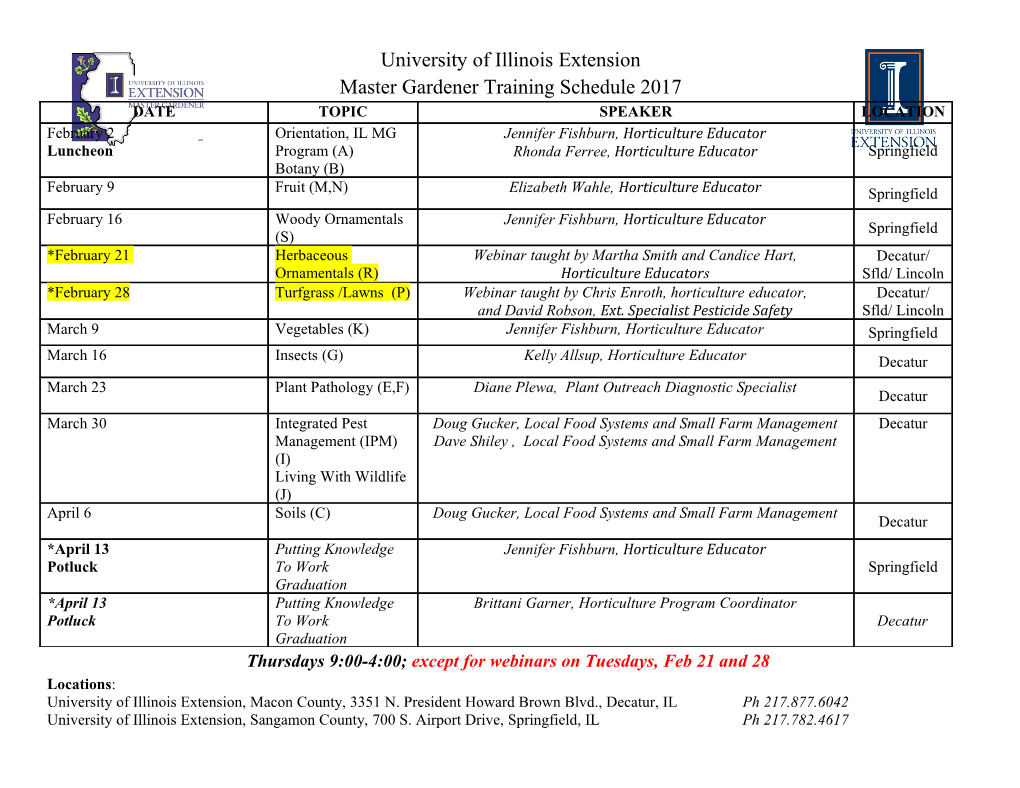
Preprint accepted at Nature Geoscience on 15th May 2020 and made available under the CC-BY-NC-ND 4.0 license. c 2020 Global distribution of sediment-hosted metals controlled by craton edge stability Mark J. Hoggard,∗,a,b Karol Czarnota,∗,c,d Fred D. Richards,a,e David L. Huston,c A. Lynton Jaques,d & Sia Ghelichkhand aDepartment of Earth and Planetary Sciences, Harvard University, USA. bLamont-Doherty Earth Observatory, Columbia University, USA. cGeoscience Australia, Canberra, Australia. dResearch School of Earth Sciences, Australian National University, Canberra, Australia. eDepartment of Earth Science and Engineering, Imperial College London, UK. ∗ www.mjhoggard.com; mark [email protected]; [email protected] Cite as: Hoggard, M.J., K. Czarnota, F.D. Richards, D.L. Huston, A.L. Jaques, & S. Ghelichkhan (2020). Global distribution of sediment-hosted metals controlled by craton edge stability, Nature Geoscience, 13(7), 504–510. https://doi.org/10.1038/s41561-020-0593-2 Sustainable development and the transition to a clean-energy economy drives ever-increasing demand for base metals, substantially outstripping the discovery rate of new deposits and necessitating dramatic im- provements in exploration success. Rifting of the continents has formed widespread sedimentary basins, some of which contain large quantities of copper, lead and zinc. Despite over a century of research, the geological structure responsible for the spatial distribution of such fertile regions remains enigmatic. Here, we use statistical tests to compare deposit locations with new maps of lithospheric thickness, which outline the base of tectonic plates. We find that 85% of sediment-hosted base metals, including all giant deposits (>10 megatonnes of metal), occur within 200 km of the transition between thick and thin lithosphere. Rifting in this setting produces greater subsidence and lower basal heat flow, enlarging the depth extent of hydrothermal circulation available for forming giant deposits. Given that mineralisation ages span the last 2 billion years, this observation implies long-term lithospheric edge stability and a genetic link between deep Earth processes and near-surface hydrothermal mineral systems. This discovery provides an unprecedented global framework for identifying fertile regions for targeted mineral exploration, reducing the search-space for new deposits by two-thirds on this lithospheric thickness criterion alone. Consumption of base metals (copper, lead, zinc and nickel) porphyry copper deposits are generated by wet melting in over the next ∼25 years is set to exceed the total produced the mantle wedge above a subducting slab, followed by em- in human history to date (Ali et al., 2017; Schodde, 2017). placement of these melts into the shallow overlying crust and Moreover, critical minerals (e.g. cobalt, indium and germa- subsequent concentration by high-temperature hydrothermal nium) are often produced as by-products of base metal min- circulation (Griffin et al., 2013). Thus, by combining the ing and are essential in many high-tech applications (Nas- plate tectonic setting with geological constraints on the loca- sar et al., 2015; Mudd et al., 2018; IRENA, 2019; Dominish tion of key mineral system ingredients, the search-space for et al., 2019; Sovacool et al., 2020). A growing concern is new magmatic deposits can be substantially reduced (Rosen- that the rate of exploitation of existing reserves is outstrip- baum et al., 2005; Butterworth et al., 2016; O’Reilly et al., ping discovery of new deposits, despite exploration expendi- 2017). ture tripling during the 2005–2012 minerals boom (Ali et al., In the case of sediment-hosted deposits, most assessments 2017; Schodde, 2017). To reverse this trend and supply the to date have focused on their genesis within the context of resources necessary to comply with policies such as the Paris Earth’s secular evolution, as well as past tectonic and geo- Climate Agreement and United Nations’ Sustainable Devel- graphic settings (McCuaig et al., 2018). The majority are opment Goals, improved techniques for locating new deposits found in failed rift and passive margin settings, and it is are required, particularly those buried under shallow sedi- generally agreed that basin-scale hydrothermal circulation is mentary cover or ice (The Uncover Group, 2012). required to scavenge sufficient metals to form giant deposits (Figure 1a; Leach et al., 2010; Hitzman et al., 2010; Manning Narrowing the search-space for new deposits & Emsbo, 2018). Metals are mobilised and transported by ◦ In mineral exploration, initial area selection at continen- oxidised brines with moderate temperatures (80–250 C) and tal scales is arguably the most important step, as successful moderate-to-high salinity (10–30 wt.% NaCl), limiting their identification of fertile regions can compensate for many sub- maximum age to the Great Oxidation Event at 2.4 Ga (Leach sequent errors (McCuaig et al., 2010). Over the last two et al., 2010; Hitzman et al., 2010). These fluids are sourced decades, the search for analogues of known deposits has pro- from evaporites at low latitudes and remain buffered as they gressed towards a more holistic determination of factors con- pass through voluminous oxidised terrestrial sediments, al- trolling deposit generation and preservation (Wyborn et al., lowing them to scavenge lead from arkosic sandstones and 1994; Bierlein et al., 2006; McCuaig & Hronsky, 2014; Den- felsic volcanics, as well as copper and zinc from mafic rocks tith et al., 2018; Skirrow et al., 2019). Mineral systems anal- (Leach et al., 2010; Hitzman et al., 2010). Transport along ysis has resulted in a growing acceptance that the spatial faults, either during rifting or basin inversion, focuses these distribution of deposits associated with magmatic processes fluids into oxidation-reduction interfaces, such as distal-facies is controlled by lithospheric-scale structure (McCuaig et al., black shales, where metals precipitate (Figures 1b and 1c; 2010; Begg et al., 2010; Griffin et al., 2013). For example, Huston et al., 2016). 1 Preprint accepted at Nature Geoscience on 15th May 2020 and made available under the CC-BY-NC-ND 4.0 license. c 2020 Figure 1: Mineralisation system for genesis of sediment-hosted base metal deposits. (a) Schematic illustration of deposit location in extensional rift settings. Basinal brines sourced from evaporites scavenge metals from oxidised terrestrial sediments and volcanics (v) on route to metal deposition sites in black shales (Manning & Emsbo, 2018). Notice variable vertical exaggeration (VE) and prominence of the lithosphere-asthenosphere boundary (LAB) edge illustrated at 1:1 scale. Schematic based on architectural con- straints from the Australian Carpenteria Zinc Belt and Polish Fore-Sudetic Block. (b) Stability field of Fe–S–O minerals as a function of temperature and redox conditions; mSO4 = molarity of sulphate; mH2S = molarity of sulphide; thick black lines = solubility of zinc (and lead) in brine, calculated for fluid salinity = 10 wt.% NaCl, total concentration of sulphur species = 10−2.5 M, and pH = 4.5 (Huston et al., 2016); blue arrow = fluid path for metal precipitation by oxidation-reduction deposition mechanism. (c) Same for copper solubility. Sediment-hosted base metal deposits are desirable due to Relationship with lithospheric structure their greater quantity of contained metal (in contrast to vol- We begin by collating global inventories of six major base- canogenic massive sulfides) and higher grades of ore (in com- metal mineral systems from published sources (Methods). parison to porphyry copper), resulting in lower environmental Three are magmatic and three are sediment-hosted, which in- et al. degradation during extraction (Dominish , 2019; Azadi clude sedimentary copper (Cu-sed), clastic-dominated lead- et al. , 2020). However, narrowing the search-space for new zinc (PbZn-CD, commonly also referred to as sedimentary sediment-hosted deposits has been less successful than for exhalative), and Mississippi Valley-type lead-zinc (Pb-Zn- ∼ magmatic mineral systems. Sedimentary basins cover 75% MVT). We next refine a method developed by Priestley & of the continental surface, and the key ingredients of evapor- McKenzie (2013) for mapping the thermal LAB from seismic ites associated with brine formation, felsic and mafic volcanic tomography, taking into consideration recent laboratory ex- rocks for sourcing metals, and organic rich shale precipita- periments concerning the effect of anelasticity on shear-wave tion sites, are widespread and do not substantially reduce velocities (Yamauchi & Takei, 2016; Methods). This bench- this search-space. The first-order geological control that lo- marking procedure is necessary in order to increase consis- calises their spatial distribution throughout the continents tency between LAB maps obtained for different tomography remains unknown, severely limiting predictive power for iden- models, which can image surprisingly variable seismic veloc- tifying new targets. A classic example comes from the Car- ities. A high resolution regional LAB map over Australia pentaria Zinc Belt in northern Australia, which contains sev- is obtained from the FR12 model (Fishwick & Rawlinson, eral world class clastic-dominated lead-zinc deposits formed 2012) and is calibrated using nine local paleogeotherms de- between 1.8–1.4 Ga (Figure 2a). These deposits lie along rived from thermobarometry of mantle peridotite xenoliths an arcuate trend that runs oblique to mapped geology
Details
-
File Typepdf
-
Upload Time-
-
Content LanguagesEnglish
-
Upload UserAnonymous/Not logged-in
-
File Pages104 Page
-
File Size-