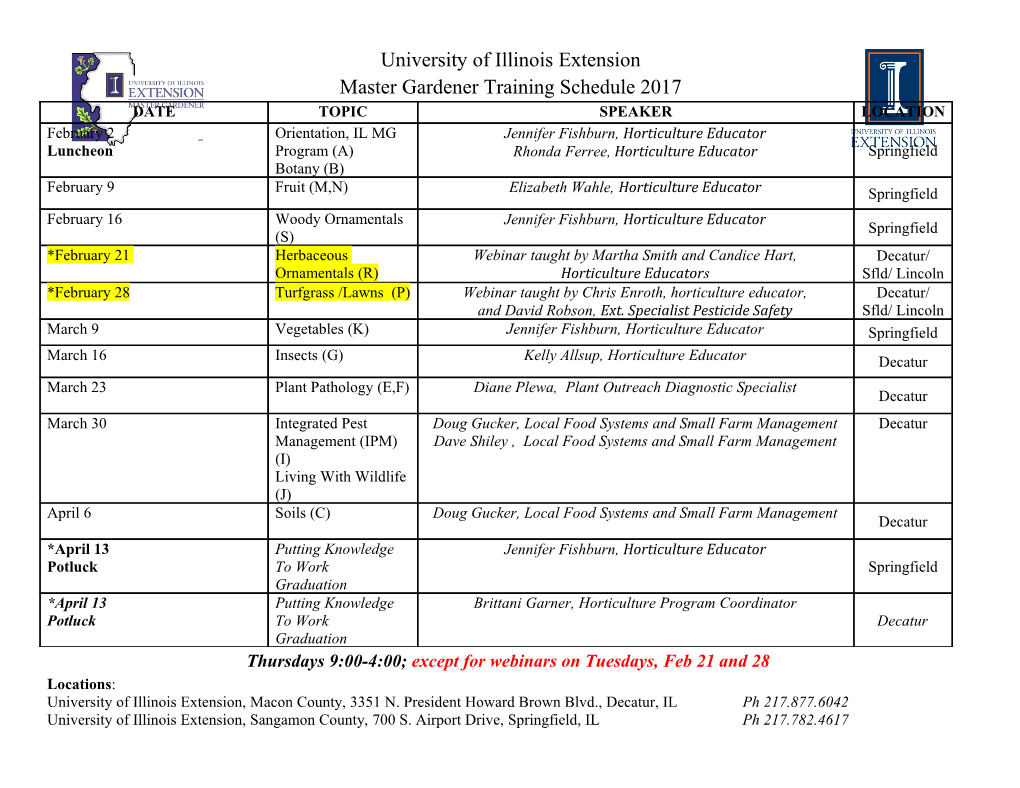
Zhang and Sagiya Earth, Planets and Space (2017) 69:82 DOI 10.1186/s40623-017-0668-5 FULL PAPER Open Access Shear strain concentration mechanism in the lower crust below an intraplate strike‑slip fault based on rheological laws of rocks Xuelei Zhang1* and Takeshi Sagiya2 Abstract We conduct a two-dimensional numerical experiment on the lower crust under an intraplate strike-slip fault based on laboratory-derived power-law rheologies considering the efects of grain size and water. To understand the efects of far-feld loading and material properties on the deformation of the lower crust on a geological time scale, we assume steady fault sliding on the fault in the upper crust and ductile fow for the lower crust. To avoid the stress singularity, we introduce a yield threshold in the brittle–ductile transition near the down-dip edge of the fault. Regarding the physical mechanisms for shear strain concentration in the lower crust, we consider frictional and shear heating, grain size, and power-law creep. We evaluate the signifcance of these mechanisms in the formation of the shear zone under an intraplate strike-slip fault with slow deformation. The results show that in the lower crust, plastic deformation is possible only when the stress or temperature is sufciently high. At a similar stress level, ∼100 MPa, dry anorthite begins to undergo plastic deformation at a depth around 28–29 km, which is about 8 km deeper than wet anorthite. As a result of dynamic recrystallization and grain growth, the grain size in the lower crust may vary laterally and as a function of depth. A comparison of the results with constant and non- constant grain sizes reveals that the shear zone in the lower crust is created by power-law creep and is maintained by dynamically recrystallized material in the shear zone because grain growth occurs in a timescale much longer than the recurrence interval of intraplate earthquakes. Owing to the slow slip rate, shear and frictional heating have negligible efects on the deformation of the shear zone. The heat production rate depends weakly on the rock rheology; the maximum temperature increase over 3 Myr is only about several tens of degrees. Keywords: Intraplate strike-slip fault, 2-D thermal-mechanical fault model, Ductile shear zone Introduction Andreas Fault (see Lachenbruch and Sass 1980) has been Ductile shear zones are believed to exist in the lower explained by shear heating in the lower crust. Te tem- crust below interplate strike-slip faults on the basis of perature anomaly in the lower crust can reach several various observational, experimental, and theoretical hundred degrees, which can create an observable heat studies as well as geological observations of exhumed fow anomaly on the surface (e.g., Tatcher and England shear zones. Termal weakening due to shear heating 1998; Leloup et al. 1999; Takeuchi and Fialko 2012). A has been considered as an important process for the large temperature anomaly can result in a weak zone with development and maintenance of shear zones (e.g., Yuen low seismic velocity that can be observed as a heteroge- et al. 1978; Fleitout and Froidevaux 1980). Observation neous velocity structure in the seismic tomography data of the broadly distributed heat fow anomaly on the San (Wittlinger et al. 1998). Furthermore, mylonite outcrops of exhumed faults (White et al. 1980) provide direct evi- *Correspondence: [email protected]‑u.ac.jp dence for the existence of ductile shear zones in the lower 1 Graduate School of Environmental Studies, Nagoya University, Nagoya, crust under interplate (e.g., Rutter 1999; Little et al. 2002) Japan Full list of author information is available at the end of the article © The Author(s) 2017. This article is distributed under the terms of the Creative Commons Attribution 4.0 International License (http://creativecommons.org/licenses/by/4.0/), which permits unrestricted use, distribution, and reproduction in any medium, provided you give appropriate credit to the original author(s) and the source, provide a link to the Creative Commons license, and indicate if changes were made. Zhang and Sagiya Earth, Planets and Space (2017) 69:82 Page 2 of 12 and intraplate faults (e.g., Shimada et al. 2004; Fusseis et al. 2006; Takahashi 2015). Compared with interplate faults, intraplate strike-slip faults have much smaller slip rates, at <1 mm/year, and their age is much younger in the Japanese Islands (less than 3 Myr; Doke et al. 2012). However, heterogeneous structures beneath intraplate strike-slip faults observed by seismic tomography (e.g., Nakajima and Hasegawa 2007; Nakajima et al. 2010) and magnetotelluric survey (e.g., Ogawa and Honkura 2004; Yoshimura et al. 2009) suggest the existence of localized weak zones in the lower crust just below intraplate active faults (Iio et al. 2002, 2004). Te spatial resolution of these observations Fig. 1 Model geometry is insufcient to resolve the structures of such ductile shear zones. Terefore, understanding the mechanisms that lead to shear strain concentration in the lower crust beneath an intraplate strike-slip is an important step in dominant mode of deformation, an infnitely long verti- understanding the deformation of the crust. cal creeping fault is assumed with the fault strike parallel In this study, we construct a series of numerical mod- to the y-axis. Te lower crust is deformed by plastic fow, els on the deformation in the lower crust below an active and there is a semi-brittle regime between the upper and intraplate strike-slip fault based on laboratory-derived the lower crust. Te lower boundary of the semi-brittle rheological laws. We simulate the evolution of viscosity regime is the brittle–ductile transition (BDT), the depth and deformation patterns of the lower crust beneath an of which depends on the assumption of crustal rheology immature intraplate strike-slip fault on a geological time- (Table 1). Considering the symmetry of the vertical strike- scale. We consider three mechanisms of strain localization: slip fault, our model region includes only one side of the shear and fault frictional heating, grain size reduction, and fault bounded by the surface and a vertical plane of bilateral power-law creep. Te efect of water is quantitatively evalu- symmetry, which is taken to be the center of the shear zone. ated with water fugacity. We discuss the role of shear strain Rheology concentration mechanisms and boundary conditions in the development of the shear zone. In addition, we compare Te constitutive relation for the plastic fow of rocks is the shear zones beneath intraplate and interplate strike-slip described as follows (e.g., Bürgmann and Dresen 2008): faults to identify the controlling factors for lower crustal − Q + pV ε˙ = Aτ nL mf r exp − , (1) shear localization under intraplate strike-slip faults. s H2O RT Model description We simulated the deformation of the lower crust beneath Table 1 Model confgurations an intraplate strike-slip fault by applying a velocity boundary condition representing far-feld loading. We Model Crustal rheology Fault type Grain size solved the stress equilibrium equation and the heat fow W1C Wet anorthite Intraplate strike-slip Constant (L 500 equation for a thermo-mechanical coupled model, and fault µm) = we used laboratory-derived rheological laws to control D1C Dry anorthite Intraplate strike-slip Constant (L 500 = the behavior of rocks. fault µm) W30C Wet anorthite Interplate strike-slip Constant (L 500 = Model geometry fault µm) W1E Wet anorthite Intraplate strike-slip Equilibrium Te model domain is 35 km thick in the vertical (z) direc- fault tion and 30 km wide in the fault-normal (x) direction. Te D1E Dry anorthite Intraplate strike-slip Equilibrium Mohorovičić (Moho) discontinuity is represented by a hor- fault izontal boundary at a depth of 35 km. Following Tatcher W30E Wet anorthite Interplate strike-slip Equilibrium and England (1998), we considered the problem in a 2-D fault plane perpendicular to the fault trace, as shown in Fig. 1. Six model confgurations were tested by this study. The rheological parameters We assumed two layers: a rigid upper crust and a ductile for anorthite are summarized in Table 2. The nomenclature of the model confguration is as follows: The frst letter denotes water content (D, dry; W, wet), lower crust, and the entire crust is composed of wet or dry and the last letter denotes grain size (C, constant; E, equilibrium). The number anorthite. In the upper crust where brittle failure is the between two letters denotes the total relative velocity in mm/year Zhang and Sagiya Earth, Planets and Space (2017) 69:82 Page 3 of 12 where τs is the maximum shear stress given by the square root of the second deviatoric stress invariant. L is the grain size. fH2O is water fugacity. Q and V are activa- tion energy and activation volume, respectively. R is the universal gas constant. p is pressure, and A, n, m, r are material constants. Te laboratory-derived parameters for anorthite are summarized in Table 2. Regarding the physical mechanism of plastic fow, in this study, we con- sidered both difusion creep and dislocation creep. For a given mineral, we assume that the same shear stress con- trols the two deformation mechanisms (e.g., Gueydan et al. 2001; Montési and Hirth 2003). Under this assump- ˙ tion, the total strain rate εtotal is expressed as the sum ˙ of the difusion creep strain rate εdiff and the strain rate ˙ caused by dislocation creep εdisl. ε˙total =˙εdiff +˙εdisl (2) Fig. 2 Contours of equilibrium grain size as a function of tempera- One can defne the efective viscosity, such that ture and stress, assuming wet anorthite rheology = τs ηeff . (3) ε˙total From this assumption, we expect a large variation in Te grain size in this study is assumed following the model grain size under the thermal and stress conditions of the proposed by Bresser et al.
Details
-
File Typepdf
-
Upload Time-
-
Content LanguagesEnglish
-
Upload UserAnonymous/Not logged-in
-
File Pages12 Page
-
File Size-