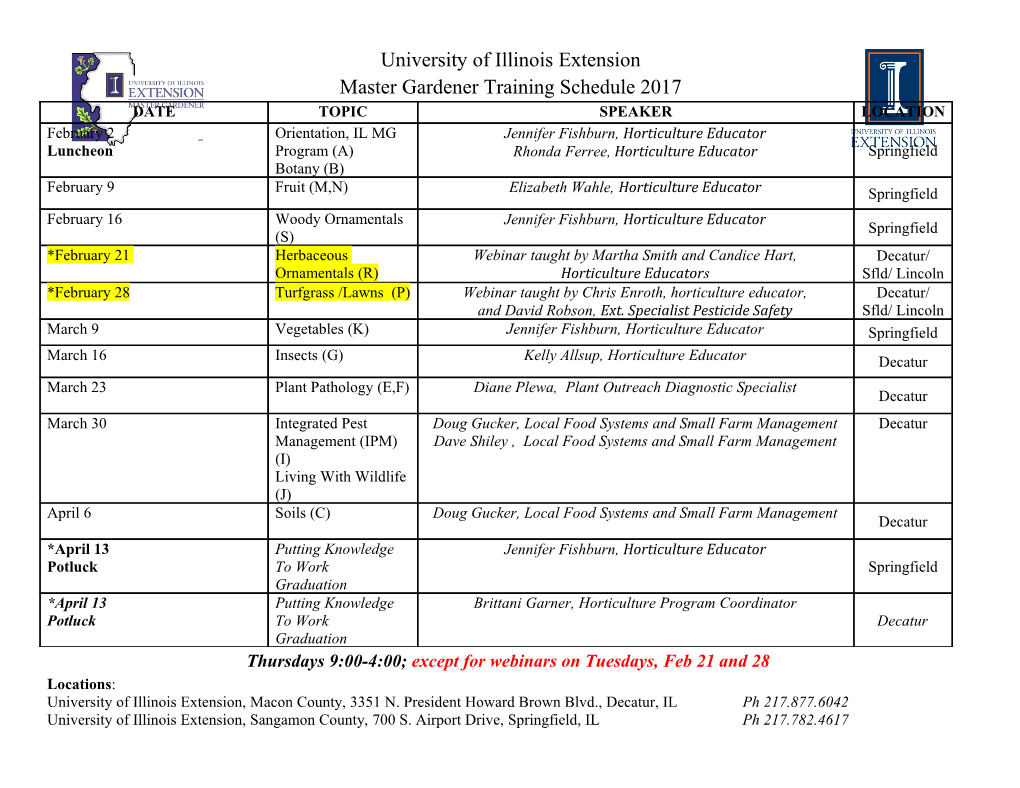
A Brief History of Space Climatology: From the Big Bang to the Present Michael Xapsos, NASA Goddard Space Flight Center NSREC 2018 Short Course Outline I. Background ................................................................................................................. 2 II. Introduction ................................................................................................................. 6 III. The Early Universe ...................................................................................................... 6 A. Big Bang Nucleosynthesis ...................................................................................... 7 B. Stellar Nucleosynthesis ........................................................................................... 8 C. Extreme Event Nucleosynthesis ............................................................................. 9 D. Abundances and Radiation Effects of the Elements ............................................. 10 IV. Transition to Modern Times ...................................................................................... 11 V. Modern Times – Space Climatology ........................................................................ 14 A. Definition of Space Climatology and Space Weather .......................................... 15 B. Galactic Cosmic Rays ........................................................................................... 16 1. Properties .......................................................................................................... 16 2. Models............................................................................................................... 20 3. Current Issue: Elevated Fluxes during “Deep” and Prolonged Solar Minima . 22 C. Solar Particle Events ............................................................................................. 23 1. Properties .......................................................................................................... 23 2. Models............................................................................................................... 25 a) Cumulative Fluence Models ......................................................................... 26 b) Worst Case Event Models ............................................................................. 30 3. Current Issue: Use of Statistical Models vs. Worst Case Observations .......... 36 D. The Van Allen Belts .............................................................................................. 37 1. Trapped Particle Motion in the Magnetosphere ................................................ 37 2. Trapped Protons ................................................................................................ 40 a) Properties ...................................................................................................... 40 b) Models........................................................................................................... 45 3. Trapped Electrons ............................................................................................. 47 a) Properties ...................................................................................................... 47 b) Models........................................................................................................... 49 c) Current Issue: The Case of the Missing Electrons ....................................... 52 E. Example Environments Including Shielding ........................................................ 54 1. Total Ionizing Dose........................................................................................... 54 2. Single Event Upset ............................................................................................ 57 VI. Summary ............................................................................................................... 59 VII. Acknowledgments................................................................................................. 60 VIII. References ............................................................................................................. 60 I-1 I. Background This manuscript is focused on space climatology - the radiation environment observed over an extended period of time at a given location, corresponding to a space mission duration and orbit. Electronic devices and integrated circuits must be designed for this climatology in order to operate reliably. The main concerns covered are three categories of high-energy particle radiations. The first is galactic cosmic rays, a comparatively low-level flux of ions originating outside of our solar system. The second is solar particle events, bursts of radiation emitted by the sun characterized by high fluxes of protons and heavy ions. The third is the Van Allen Belts, primarily protons and electrons trapped by the Earth’s magnetic field. The plasma environment and spacecraft charging effects will not be discussed here. However, an excellent Short Course presentation of this topic was given in 2015 [Ma15]. In order to have successful, cost-effective designs and implement new space technologies, the climatology must be understood and accurately modeled. Underestimating radiation levels leads to excessive risk and can result in degraded system performance and loss of mission lifetime. Overestimating radiation levels can lead to excessive shielding, reduced payloads, over-design and increased cost. The pervasive use of commercial-off-the-shelf (COTS) microelectronics in spacecraft to achieve increased system performance must be balanced by the need to accurately predict their complex responses in space, which begins by understanding the environment in which they function. This section gives a top level description of the basic radiation effects and introduces the metrics that describe them. There are three categories of effects that are considered – single event effects (SEE), total ionizing dose (TID) and total non-ionizing dose (TNID) or displacement damage. The following manuscripts in this Short Course provide much greater detail of these effects on electronic devices and systems. SEE can be defined as any measureable effect in a circuit caused by a single incident particle. It can be either non-destructive such as single event upset (SEU) and single event transients (SETs) or destructive such as single event latchup (SEL) and single event gate rupture (SEGR). SEE can be caused by direct ionization as the incident particle passes through a sensitive region of a device, as shown in figure 1 [Ba13]. This is usually the case for incident heavy ions. The metric used to characterize the incident ion is Linear Energy Transfer (LET). LET is the ionizing energy lost by the ion per unit path length in the material of the sensitive region. One form LET units take is MeV/cm. However, it turns out that the general expression for LET is directly proportional to the material density. That dependence can be removed by dividing by the density in units of mg/cm3, giving LET units of MeV-cm2/mg. This is the form of LET most often used by the radiation effects community. This can also be viewed as the energy lost per unit path length, where path length is an areal density. The metric used to calculate single event rates in space is the charge collected at the sensitive volume junction, Q. Q = C · LET · s (1) where s is the ion’s path length through the sensitive volume and C is a unit conversion. I-2 Figure 1. SEE caused by direct ionization [Ba13]. SEE can also be caused by nuclear reaction products from the interaction of the incident particle with a nucleus in the device material. This is usually the case for incident protons and always the case for incident neutrons. For this situation the relevant metric for single event rate calculation remains the charge collected in the sensitive volume but the calculation is more complex because there can be several emerging reaction products that deposit charge. Total dose effects in electronic and photonic parts are cumulative, long-term degradation due to ionizing or non-ionizing radiation - mainly primary protons and electrons but secondary particles arising from interactions between these primary particles and spacecraft materials can also contribute. For the case of TID, the concern is mainly its effects in insulating regions of metal-oxide semiconductors (MOS) and bipolar devices, most commonly composed of SiO2. In MOS devices, ionizing radiation causes threshold voltage shifts due to exposure of gate oxides, shown in figure 2 [Sc02]. The effect first appears as parametric degradation and can eventually result in functional failure. Ionizing radiation can also cause leakage currents in MOS devices due to exposure of field oxide regions used for isolation and result in increased power consumption. Eventually the effect becomes so pronounced the transistor cannot be switched to the off state. For bipolar devices loss in performance is caused by gain degradation and increased leakage currents. The TID metric is the ionizing energy deposited per unit mass of material in the sensitive volume. It is given by I-3 TID = C · LET · ϕ (2) where ϕ is the incident particle fluence in cm-2 and C is a unit conversion. The MKS units for TID are the Gray (Gy), where 1 Gy = 1 J/kg. The cgs units are the rad, where 1 rad = 100 erg/g. Figure 2. Illustration of the TID effect in the gate region of an MOS device [Sc02]. The effects of TNID or displacement damage dose are also cumulative effects
Details
-
File Typepdf
-
Upload Time-
-
Content LanguagesEnglish
-
Upload UserAnonymous/Not logged-in
-
File Pages65 Page
-
File Size-