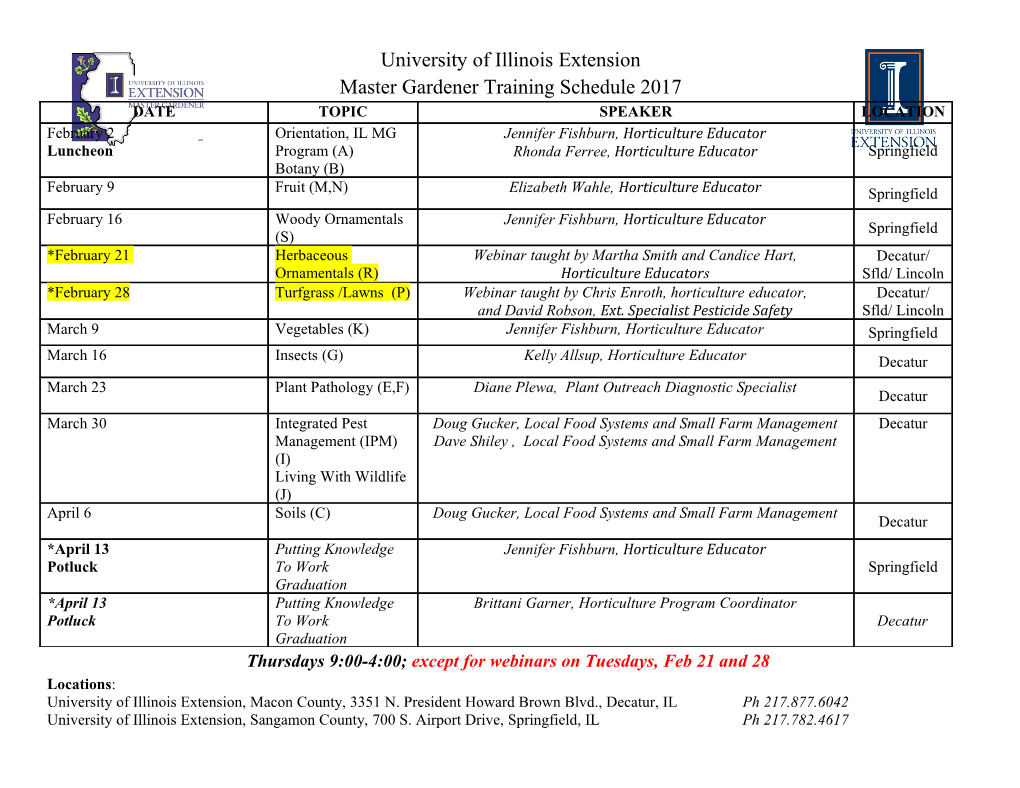
MEMBRANE PROTEIN FOLDING: THE ROLE OF AMINO ACID SEQUENCE IN SPECIFYING THE STABILITY AND CONFORMATION OF TRANSMEMBRANE OLIGOMERS by Abigail E. Kroch A dissertation submitted to The Johns Hopkins University in conformity with the requirements for the degree of Doctor of Philosophy Baltimore, Maryland February 2006 © Abigail E. Kroch 2006 All rights reserved ABSTRACT Proteins carry out essential processes of the cell, and to function properly they must adopt and maintain their native fold. Both thermodynamic and structural data for soluble protein have provided insight into the forces that drive folding in an aqueous environment. However, structural and energetic characterization for membrane proteins has lagged behind that of soluble proteins. Therefore, membrane protein model systems have been extremely valuable to understand the interactions that stabilize and specify membrane protein conformation. Early thermodynamic studies on membrane proteins demonstrated that interactions between helices promote and specify the native fold; and a large scale mutagenesis of a transmembrane dimer, Glycophorin A (GpA), showed that the amino acid sequence on a single face of the helix conferred stability to the dimer. When the NMR structure was solved, the motif highlighted by mutagenesis was found at the dimer interface. Therefore, preferential interactions at the dimer interface may stabilize and specify the native fold of a membrane protein. When this thesis study was commenced, interactions in a single sequence motif were thought to provide the driving force for association in the GpA dimer. However, in our studies, we have demonstrated that this motif, albeit critical for strong dimerization, is neither necessary nor sufficient to drive association. This work highlights that the entire sequence context provides the framework for the stability and specificity of transmembrane helix-helix interactions. The correlation of structural models to energetic measurements strongly suggests that local ii packing defects may be responsible for the perturbation upon amino acid substitution. However, upon experimental examination of structural changes, packing defects may also initiate global conformation change in the dimer and therefore these mutations would perturb interactions between the helices and between the helices and solvating lipids. The information derived from these studies on a model membrane protein dimer was also applied to transmembrane segments that are involved in vesicle fusion. The association of these proteins, syntaxin and synaptobrevin, may be essential to the process of fusion. Both homo-dimerization and hetero-dimerization of these proteins is weak but is still modulated by the amino acid sequence. In this case, a dynamic equilibrium for protein-protein interactions may be critical for biological function. Therefore, amino acid sequences can encode both stable and transient protein interactions that are biologically relevant. Thus, when we study membrane protein structure and interactions, it is necessary to consider the forces that both drive and repel folding, since it is the equilibrium of these forces that establishes biologically functional proteins. Thesis Advisor: Dr. Karen G. Fleming Secondary Reader: Dr. Bertrand Garcia-Moreno Thesis Committee: Dr. George Rose Dr. Joel Schildbach iii To my Opa, Morris Adolph Kroch, Whose courage and strength allowed my very existence. iv ACKNOWLEDGEMENTS This work would not have been possible without the help and support of my friends and family. I thank my mother and father for their encouragement and endorsement of everything I have done, and for reminding me that the most important thing is the work, and especially for my father’s advice and assistance in the completion of this thesis. I thank my sisters, Miriam and Deborah, who are always available for support, encouragement, and a distracting conversation. And, my nieces Katie and Sarah, and my nephew Jake, who provide endless entertainment and remind me of the things that are truly important. My grandfather and my late grandmother, Sam and Jane Burgess, who have always demonstrated their pride and support through the years. To all my friends, for without them, I could not have made it through, and especially could not have had so much fun in graduate school. I especially thank, Eric Davison, who is always willing to feed me, listen to me, and support me, more than I could truly deserve. I thank Alex Ebie, my labmate and confidant, who has helped me through the most trying times. I also thank my sister moon, Kittiya Lee; Michelle Beaucher, Prashanth Rangan, Naveen Michaud, Katherine Tripp, Mark Zweifel, Naomi Courtemanche, Guru Thuduppathy, Timo Street, Judy Gerring, Kate Murphy, Scott Jackson, Susu Hunniecutt, Youjin Lee, Alana McGill, Kedy’ky Sherrill, and her dear grandmother Dessie Mae Tyler, who I will always miss; and, all the members of the Biology class that entered in 2000. I also thank the Midtown Yoga Studio, and all the v teachers there for their support and help in the advancement in my yoga practice, which undoubtedly helped in the completion of this work. I would like to thank the faculty of the Biology and Jenkins departments for their mentorship and support over the years. I thank Allen Shearn, who acted as my advocate; Doug Barrick, a wonderful mentor, karaoke singer, and an inspiration as a scientist. I especially thank the members of my committee, Bertrand Garcia-Moreno, Neil Clarke, and George Rose for being supportive, and providing me with sound advice and creative ideas. I thank all the support staff at Hopkins, especially Jerry Levin, Joan Miller, and Ken Rutledge, and all the staff of the Biology and Jenkins Departments, the guys in the Mudd Stockroom, the delivery guys, and the housekeeping staff. I especially thank Deborah DiLazzero, who has been instrumental in all my recent achievements. I must thank all the members of the Fleming Lab, who have made coming to work stimulating and fun, Ann Marie Stanley, who has been my partner in crime over the years and always up for a stimulating scientific conversation; Felix Kobus, our former post doc; and the newer members of our lab, Alex Ebie, Nancy Burgess, and Preston Moon, who keep the lab a fun place to work. I also thank our former technicians, Matt Eisley and Maridel Lares; and all former undergraduates, especially Yasuko Takeda, and all former rotation students. Finally, I must thank my advisor, Dr. Karen G. Fleming, who taught me an analytical approach to biological problems, got me excited about studying biophysics and gave me the confidence to pursue it. Karen has given me the support and the freedom to become a confident and independent scientist. vi TABLE OF CONTENTS Abstract ii Acknowledgments v Table of Contents vii Abbreviations x List of Tables xi List of Figures xii CHAPTER 1. Introduction 1 1.1 Overview and Perspectives 1 1.2 Forces that drive membrane protein folding 4 1.3 A thermodynamic approach to membrane protein folding 8 1.4 Relationship between sequence and stability 18 1.5 The relevance to membrane protein stability in the cell 21 1.6 Amino acid sequence specifies stable helix-helix interactions 27 1.7 Thermodynamic measurements for membrane protein stability 32 1.8 Thesis Overview 35 CHAPTER 2. Sequence context modulates the stability of a GxxxG mediated transmembrane helix-helix dimer 39 2.1 Summary 39 vii 2.2 Introduction 40 2.3 Materials and Methods 42 2.4 Results 46 2.5 Discussion 56 2.6 Conclusions 61 CHAPTER 3. Complex interactions at the helix-helix interface stabilize the Glycophorin A transmembrane dimer 63 3.1 Summary 63 3.2 Introduction 64 3.3 Materials and Methods 67 3.4 Results 71 3.5 Discussion 86 3.6 Conclusions 92 CHAPTER 4. Packing defects in the Glycophorin A transmembrane dimer induce global rearrangements 93 4.1 Summary 93 4.2 Introduction 94 4.3 Materials and Methods 98 4.4 Results 101 4.5 Discussion 120 4.6 Conclusions 125 viii CHAPTER 5. Alternate interfaces may mediate homomeric and heteromeric assembly in the transmembrane domains of SNARE proteins 126 5.1 Summary 126 5.2 Introduction 128 5.3 Materials and Methods 132 5.4 Results 135 5.5 Discussion 150 5.6 Conclusions 154 CHAPTER 6. Conclusions and Remarks 156 REFERENCES. 159 VITA. 171 ix ABBREVIATIONS GpA (Glycophorin A) BR (Bacteriorhodopsin) TM (transmembrane domain) C8E5 (Polyoxyethylene 5 octyl ether) C14 (3-(N,N-Dimethylmyristylammino)propanesulfonate) SDS-PAGE (sodium dodecyl sulfate polyacrylamide gel electrophoresis) CuOP (copper phenanthroline) DTNB (5,5'-dithio-bis(2-nitrobenzoic acid)) AUC (Analytical Ultracentrifugation) SNARE (soluble NSF attachment protein receptors) SN (Staphylococcal aureus Nuclease) Syx (Syntaxin 1A) Syb (Synaptobrevin 2 aka VAMP2) x LIST OF TABLES CHAPTER 3. Table 3.1 Double Mutant Analysis of GpA TM 72 Table 3.2 Alignment of experimental constructs that test the role of 81 the GxxxG motif CHAPTER 4. Table 4.1 Analysis of structural models generated by CHI. 116 CHAPTER 5. Table 5.1 Free energy of association for syntaxin and synaptobrevin 136 constructs. xi LIST OF FIGURES CHAPTER 1. Figure 1.1 The crystal structure of Bacteriorhodopsin. 10 Figure 1.2 The two-stage model for membrane protein folding. 12 Figure 1.3 The thermodynamic cycle for single point substitutions 17 in a transmembrane α-helical dimer. Figure 1.4 The GpA TM dimer NMR structure. 22 Figure 1.5 The ToxR assay measures dimer affinity in vivo 25 CHAPTER 2. Figure 2.1 The free energy perturbations due to mutations at 47 the protein-protein interface of the GpA TM. Figure 2.2 Equilibrium distributions of GpA TM dimer mutants. 49 Figure 2.3: A linear correlation between parameterized structural 52 features and free energy perturbation. Figure 2.4: Contributions of each of the structure-based energy 54 terms to the of ∆∆Gcalc sum. CHAPTER 3. Figure 3.1 Relative dimer population of selected GpA TM mutants. 74 Figure 3.2 The free energy of coupling for alanine double mutants.
Details
-
File Typepdf
-
Upload Time-
-
Content LanguagesEnglish
-
Upload UserAnonymous/Not logged-in
-
File Pages187 Page
-
File Size-