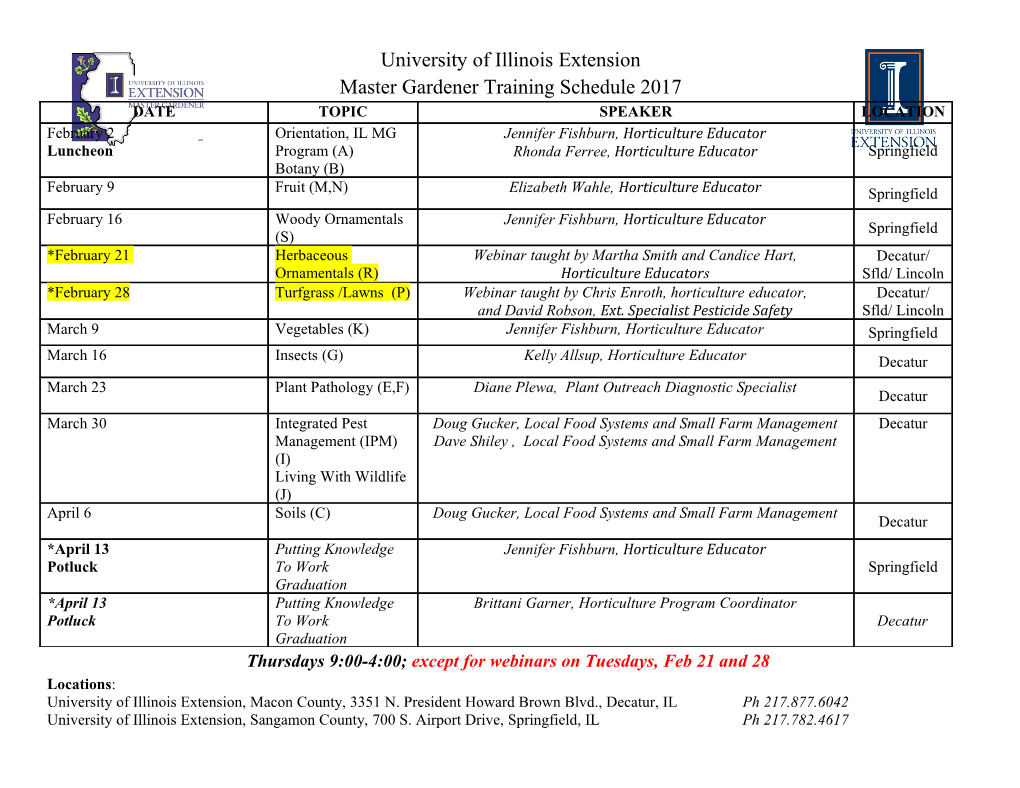
ARTICLES https://doi.org/10.1038/s41561-017-0006-3 Oxygenation as a driver of the Great Ordovician Biodiversification Event Cole T. Edwards1*, Matthew R. Saltzman2, Dana L. Royer3 and David A. Fike4 The largest radiation of Phanerozoic marine animal life quadrupled genus-level diversity towards the end of the Ordovician Period about 450 million years ago. A leading hypothesis for this Great Ordovician Biodiversification Event is that cooling of the Ordovician climate lowered sea surface temperatures into the thermal tolerance window of many animal groups, such as corals. A complementary role for oxygenation of subsurface environments has been inferred based on the increasing abundance of skeletal carbonate, but direct constraints on atmospheric O2 levels remain elusive. Here, we use high-resolution paired bulk car- bonate and organic carbon isotope records to determine the changes in isotopic fractionation between these phases throughout the Ordovician radiation. These results can be used to reconstruct atmospheric O2 levels based on the O2-dependent fraction- ation of carbon isotopes by photosynthesis. We find a strong temporal link between the Great Ordovician Biodiversification Event and rising O2 concentrations, a pattern that is corroborated by O2 models that use traditional carbon–sulfur mass balance. We conclude that that oxygen levels probably played an important role in regulating early Palaeozoic biodiversity levels, even after the Cambrian Explosion. uring the Ordovician period one of the greatest biological eses include increased silicate weathering12 and the advent of land 13 radiations of the Phanerozoic took place, when genus-level plants ), but a role for increased atmospheric O2 is possible via an Ddiversity quadrupled and ecospace utilization increased1. increase in total atmospheric pressure and the associated inhibi- During this Great Ordovician Biodiversification Event (GOBE), tion of solar optical depth, scattering incident solar radiation that marine communities expanded into new niches such as epifaunal would have otherwise contributed to the surface latent heat flux14. suspension feeding and deep burrowing2. This new niche space was These arguments for linking oxygenation to cooling and biodiver- largely exploited by members of the Palaeozoic Evolutionary Fauna sification, while compelling, are hindered by poorly constrained (EF), which includes articulated brachiopods, crinoids, ostracodes, ocean–atmosphere oxygen records. Existing isotope mass balance cephalopods, corals, and bryozoans1. Both the Cambrian EF (trilo- models are hampered by coarse time resolution (typically 10 Myr bites and inarticulate brachiopods) and Modern EF (bivalves, gas- bins) that are not capable of resolving changes in atmospheric O2 as tropods, fish, and so on) also diversified, but it was the expansion of a cause of the main pulses of biodiversification across the GOBE15–17 the Paleozoic EF that drove the GOBE. (Supplementary Fig. 1). The absence of charcoal is interpreted to 18 The causes of the GOBE remain poorly understood and may reflect atmospheric O2 levels below 13–15% until the Late Silurian , include both intrinsic biological factors and external environmen- but primitive non-vascular land plants only expanded into terres- tal drivers (such as global cooling, nutrient delivery from erosion, trial environments by the Middle–Late Ordovician13, thus the char- higher sea levels that expanded habitable platform area, and oxygen- coal record is not well suited to constrain Early Ordovician O2 levels. ation)1,3–5. Oxygen isotopes from well-preserved conodont apatite Land plant expansion is thought to have increased organic burial provide proxy evidence for high sea surface temperatures (~40 °C) rates and oxygenated the atmosphere to near modern levels13, but the at the onset of the Ordovician that may have inhibited diversifica- timing and magnitude of this oxygenation is also poorly resolved. tion, but global cooling throughout the Early–Middle Ordovician Similarly, although iron-based redox proxies suggest that O2 levels brought temperatures closer to modern conditions and possibly into were between 2% and 21% throughout the Ordovician, their reso- the tolerance window (27–32 °C) for members of the Palaeozoic EF6. lution is not yet sufficient to resolve finer temporal trends19. Here Cooling oceans could also store more dissolved oxygen and more we apply a new approach to reconstruct the changes in atmospheric effectively ventilate subsurface environments, which would in turn oxygen with high age-resolution based on the O2-dependence of create a stronger vertical gradient in carbonate saturation that low- carbon isotope fractionation during photosynthesis20. ered the metabolic costs of skeletal carbonate biomineralization 7 8 in surface waters . A global increase in atmospheric oxygen and Effects of atmospheric O2 on photosynthesis oxygenation of shallow marine environments may have also eased Our estimates for Ordovician atmospheric O2 are based on the link stressful conditions for benthic animal life9 and expanded the range between photosynthetic fractionation of stable carbon isotopes in of habitable ecospace for infaunal burrowers deeper into the sedi- primary producers (such as marine phytoplankton) and changing O2. ment10. A more oxygenated ocean could also have supported more The carbon fixation enzyme ribulose 1,5-bisphosphate carboxyl- predators in the food chain (fish and cephalopods), setting into ase–oxygenase (Rubisco) has dual carboxylase/oxygenase func- motion an evolutionary ‘arms race’11. Ordovician global cooling is tions, which results in variations in photosynthetic fractionation 20–22 generally thought to have been caused by decreasing atmospheric (εp) as a function of CO2 and O2 concentrations inside the cell . CO2 (the cause of this drop is itself not well understood, but hypoth- Atmospheric O2 in the past can thus be reconstructed if εp can be 1Department of Geological and Environmental Sciences, Appalachian State University, Boone, NC, USA. 2School of Earth Sciences, The Ohio State University, Columbus, OH, USA. 3Department of Earth and Environmental Sciences, Wesleyan University, Middletown, CT, USA. 4Department of Earth and Planetary Sciences, Washington University in St. Louis, St. Louis, MO, USA. *e-mail: [email protected] NatURE GeoscIENCE | VOL 10 | DECEMBER 2017 | 925–929 | www.nature.com/naturegeoscience 925 © 2017 Macmillan Publishers Limited, part of Springer Nature. All rights reserved. ARTICLES NATURE GEOSCIENCE reasonably estimated and the influence of changing CO2 concentra- Although circulation patterns are poorly constrained for the epei- tions on εp is accounted for. ric sea from which a portion of the samples used in this study We estimate εp from paired carbon isotopic measurements of were collected, strong upwelling systems analogous to the modern 13 13 28 carbonate rocks (δ Ccarb) and bulk organic matter (δ Corg), which Peruvian margin , if present, were probably confined to the western 29 serve as proxies for the dissolved inorganic carbon (DIC) reservoir Laurentian continental margin . Nonetheless, we consider our εp 23 13 (δd) and average phytoplankton biomass (δp), respectively (Fig. 1; record a first-order approximation. The large number of paired δ C Supplementary Table 2). These data come from a range of geologic measurements used here (n = 945) permits the use of a locally settings, including the Great Basin (western United States), North weighted smoothing line (LOESS, α = 0.2) to capture the overall American midcontinent, eastern North America and Anticosti trend in εp-estimated, which increased from about 20.2‰ during the Island (Canada), and Argentine Precordillera. Biases associated Early Ordovician to 22.5‰ during the middle Late Ordovician with gradients in seawater δ 13C or isolated populations of phyto- (Supplementary Fig. 3). plankton with site-specific algal growth rates or physiologies are It may be possible that this pattern of increasing εp is a function minimized when compiled using data from a range of ocean basins of biologic controls (such as variations in the dominant phytoplank- and environments to highlight long-term trends. The estimated ton populations, productivity and growth rates, cell size) rather isotopic fractionation during photosynthesis in phytoplankton than environmental changes. This interval spans the ‘Ordovician 24 (εp-estimated) can be expressed as : Plankton Revolution’, a period recognized for the diversification of several plankton groups30, but the poor fossil record of Ordovician phytoplankton (that is, acritarchs) makes it difficult to assess the ɛ=pe− stimated(‰)( δ+dp1, 000)∕δ +−1, 000 11 × ,000 (1) () true diversity of algae. However, considering that this overall εp increase occurred over several million years and is reproducible 13 To estimate δd we adjust δ Ccarb for fractionation effects associ- across several basins, we infer that local biologic factors were not 6 ated with temperature (using estimates from the literature ) and as important as ambient CO2 and O2 levels, which are more likely the formation of carbonate in seawater (Supplementary Fig. 7; to affect the carbon cycle globally. Thus, an increase in εp can best see Supplementary Information for calculations, a discussion of be explained by some combination of either rising atmospheric CO2 corrections, and sensitivity testing for constant and alternative or O2 because both molecules directly impact isotopic fractionation 13 temperature estimates).
Details
-
File Typepdf
-
Upload Time-
-
Content LanguagesEnglish
-
Upload UserAnonymous/Not logged-in
-
File Pages6 Page
-
File Size-