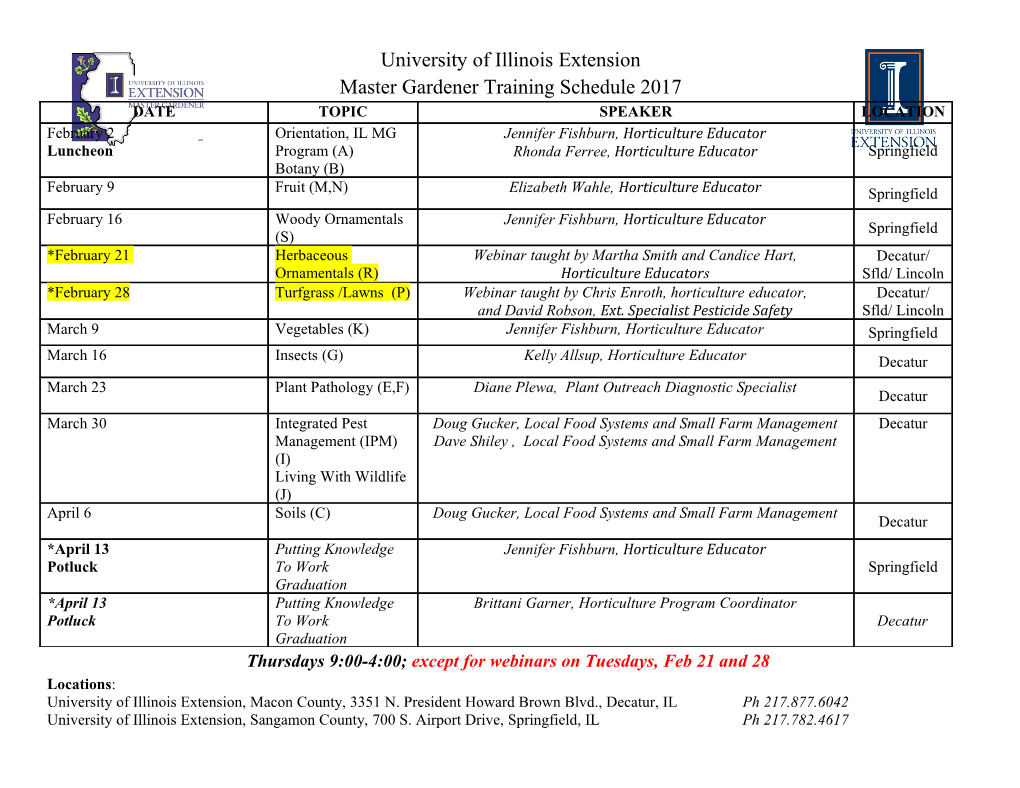
1 Ontogenetic shifts in oxygen uptake in Common Mudskipper (Periophthalmus kalolo) and 2 its role in microhabitat selection 3 4 JENNIE S. SANDBERG, AND WAYNE A. BENNETT 5 6 (WAB) The University of West Florida, Department of Biology; and (DJS) University of Essex, 7 Department of Biological Sciences 8 Running head: Oxygen uptake of Common Mudskipper 9 Manuscript type: Shorter Contribution 10 Key words: amphibious fish, metabolism, air-breathing fish 11 12 1 13 The Common Mudskipper, Periophthalmus kalolo, (family Gobiidae) is a tropical, 14 amphibious fish capable of utilizing both air and water as a respiratory medium. Although 15 little is known about their early life history, post-metamorphose fish ≤ 2.0 cm in length are 16 thought to be more dependent on tidepools than larger fish. We quantified oxygen uptake in 17 water and air of fish with standard lengths between 1.0 and 10.3 cm to identify size-related 18 shifts in aquatic and aerial oxygen consumption rates. Common Mudskippers 4.0 cm or less 19 in standard length exhibited aquatic mass-adjusted metabolic rates nearly twice those 20 measured for larger fish. Aerial mass-adjusted oxygen uptake in small mudskippers (≤ 2.0 21 cm) was ten-fold higher than values estimated for larger fish. Furthermore, air:water 22 metabolic rate ratios showed that emerged mudskippers ≤ 2.0 cm in length consumed seven 23 and one-half times more oxygen than when submerged. We argue that tidepool dependence 24 of small Common Mudskippers is linked to the markedly high metabolic demand while on 25 land, and not an inability to effectively extract oxygen from air. Elevated aerial metabolic 26 costs likely result from differing demands in air and water for physiological processes such 27 as evaporative water loss and ammonia excretion. 28 2 29 The Common Mudskipper, Periophthalmus kalolo, (family Gobiidae) is an air-breathing 30 fish common to mangal (mangrove biome) habitats throughout the Indo-Pacific (Note: 31 common and scientific names cited throughout the text follow taxonomic clarifications 32 proposed by Graham, 1997). Small post-metamorphose fish less than 2.0 cm standard length 33 prefer shaded tidepools in upper intertidal zones near mangrove stands; whereas larger 34 individuals aggregate in exposed lower intertidal mudflats (Frith, 1977; Tytler & Vaughan, 35 1983) where they may spend as much as 90% of their time out of water (Gordon et al., 1978; 36 Gordon et al., 1985). Both Brillet (1976) and Tytler and Vaughn (1983) report that at low 37 tide, small Common Mudskippers inhabiting mangals in Madagascar and Kuwait Bay, 38 respectively, never stray far from tidepools or at least wet mud, and small fish placed in the 39 open preferentially move away from exposed areas toward wet, shaded zones (Tytler and 40 Vaughn, 1983). 41 Factors influencing size-dependent distribution of Common Mudskippers among mangal 42 microhabitats are not entirely clear. Tamura and co-workers (1976) suggested that recently 43 post-metamorphic mudskippers are more effective at extracting oxygen from water than air, 44 and must therefore remain closely associated with pool environments. However, histological 45 examination has shown that epidermal vascularization in at least one periophthalmid, 46 Shuttles Hoppfish (Periophthalmus modestus), is well-developed only 10 days post-hatch 47 and should be capable of supporting cutaneous aerial respiration (Suzuki and Hagiwara, 48 1995). Likewise, Clayton (1993) has argued that high skin to gill area ratios in smaller 49 mudskippers should support a greater dependence on cutaneous rather than branchial 50 respiration leaving them better suited to aerial habitats than large fish. While numerous 51 studies have assessed aquatic or aerial oxygen uptake in mudskippers larger than 5 cm 3 52 standard length (Ip et al., 1991; Kok et al., 1998; Takeda et al., 1999; Taylor et al., 2005), no 53 study to date has quantified metabolic rates in smaller fish, leaving questions of relative 54 oxygen extraction rate unanswered. 55 In the present study, we estimate aerial and aquatic oxygen uptake of Common 56 Mudskippers to identify possible size-related metabolic shifts. In addition, we express 57 oxygen uptake in air and water as a ratio to assess relative differences between aerial and 58 aquatic respiration across size classes. Finally, we interpret metabolic rate data relative to 59 environmental and physiological constraints to better understand factors influencing activity 60 and distribution patterns of Common Mudskipper between upper and lower mangal zones. 61 62 MATERIALS AND METHODS 63 Common Mudskippers were collected between June and August 2005 from a mangal 64 habitat on the northwest edge of Hoga Island (05° 27.53′S, 123° 46.33′E) in the Wakatobi 65 National Marine Park, Sulawesi, Indonesia (Fig. 1). Fish larger than 2 cm were collected 66 from open lower mangal mudflat areas, whereas smaller fish were always captured from 67 upper mangal pools near mangrove trees. Diel air temperatures ( 0.5 C) from shaded upper 68 mangal and exposed lower mangal capture sites were measured using iButton® temperature 69 loggers (Thermocron® model DS1921H). Temperatures were recorded at 20 min intervals 70 from 28 June to 24 July 2005. 71 Captured fish were taken to Hoga Marine Research Center and transferred to covered, 72 plastic holding tanks (121 39.6 30.8 cm) containing beach sand covered with 33 ‰ 73 seawater. Emergent palm frond and driftwood pieces allowed fish free movement between air 74 and water. Holding tank temperatures were kept between 25 and 28 C with seawater changed 4 75 twice daily and sand changed every 2-3 days to maintain habitat quality. Mudskippers were 76 fasted for 48 h before respirometry trials. Each fish was used in only one aerial or aquatic 77 experiment and all fish were released to their site of capture at the end of the study. 78 Manometric respirometry (Bicudo and Johansen, 1979; Cech, 1990; Taylor et al., 2005) 79 was used to estimate total resting routine metabolic rates in air for 117 Common 80 Mudskippers. The respirometer design was similar to that of Taylor et al. (2005) and 81 consisted of a 20-mL (fish ≤ 3.0 cm standard length), or 250-mL (fish ≥ 3.1 cm) glass 82 respirometer flask connected by a manometer tube to a reference flask of equal volume. 83 Seawater-saturated blotter paper maintained high humidity levels in both flasks, and a strip of 84 10% sodium hydroxide-saturated Whatman paper attached to the respirometer flask stopper 85 removed expired carbon dioxide. An identical control (without fish) was run simultaneously 86 during each trial to correct for non-fish respiration. 87 Prior to each trial, the apparatus was pressure-tested to identify and eliminate system 88 leaks and a randomly selected fish placed into the respirometry flask. Fish were allowed to 89 adjust to the flask environment for 45 min at the experimental set point temperature at which 90 time, the respirometer was sealed and the trial begun. As oxygen was consumed, respirometer 91 flask pressure decreased causing manometer fluid displacement proportional to oxygen 92 uptake. Manometer fluid was returned to its starting level by adding air slowly and in small 93 amounts to the respirometer flask via a 10 cc Hamilton® gas-tight syringe. Measurements of 94 total injected air were recorded every 30 min for two hours with total oxygen consumption 95 (mg h-1) estimated as milligrams of injected air added divided by total trial time. 96 Estimates of total aquatic resting routine oxygen uptake were made for 92 Common 97 Mudskippers via flow-through respirometry techniques (Cech, 1990). For each trial, a fish 5 98 was randomly selected from the holding tank and transferred into 25 mL (fish ≤ 3.00 cm 99 standard length) or 250 mL (fish ≥ 3.01 cm) glass respirometer flask. Filtered seawater was 100 pumped through the respirometer flask at rates between 3 and 20 mL min-1. Flow rates varied 101 with fish mass so that oxygen content of water leaving the flask never fell below 15% of total 102 inflow oxygen concentration (Cech, 1990). Fish were allowed to adjust to the flask 103 environment for 45 min, after which three respirometer outflow water samples were collected 104 in biological oxygen demand (BOD) bottles. Bottles were filled slowly from the bottom and 105 allowed to overfill at least two times their volume to minimize the chance of atmospheric 106 oxygen uptake (Cox, 1990). Oxygen concentration of each BOD sample was determined 107 using standard Winkler titration techniques (Cox, 1990). A control flask, identical in all 108 respects but containing no fish, was included in each trial to allow correction for oxygen 109 consumption from non-fish sources. Total oxygen uptake (mg h-1) was determined as the 110 mean difference in oxygen content (mg L-1) between water leaving the control flask and 111 respirometry flask, multiplied by the respirometer flow rate (L h-1). 112 Aerial and aquatic respirometers were held at a constant temperature by submerging each 113 system into a temperature controlled, recirculating water bath maintained at 26.5 ± 0.09 C. 114 The experimental temperature was based on the average temperatures encountered in Hoga’s 115 mangal habitat. Fishes were carefully observed during experiments, and trials in which 116 individuals exhibited more than intermittent movements were omitted. At the end of each 117 trial, mudskippers were weighed (wet mass ± 0.001 g), measured (standard length ± 0.01 118 mm), and returned to a second holding tank. 119 Mass-adjusted oxygen consumption rates were calculated as the total oxygen consumed 120 (mg h-1) divided by wet mass raised to the power of 0.80. The mass adjustment exponent of 6 121 0.80 is commonly used in fish respirometry to correct for allometric relationships between 122 mass and metabolic rate (Winberg, 1956) and replaces the practice of dividing total metabolic 123 rate by mass alone (see discussion by Cech, 1990).
Details
-
File Typepdf
-
Upload Time-
-
Content LanguagesEnglish
-
Upload UserAnonymous/Not logged-in
-
File Pages19 Page
-
File Size-