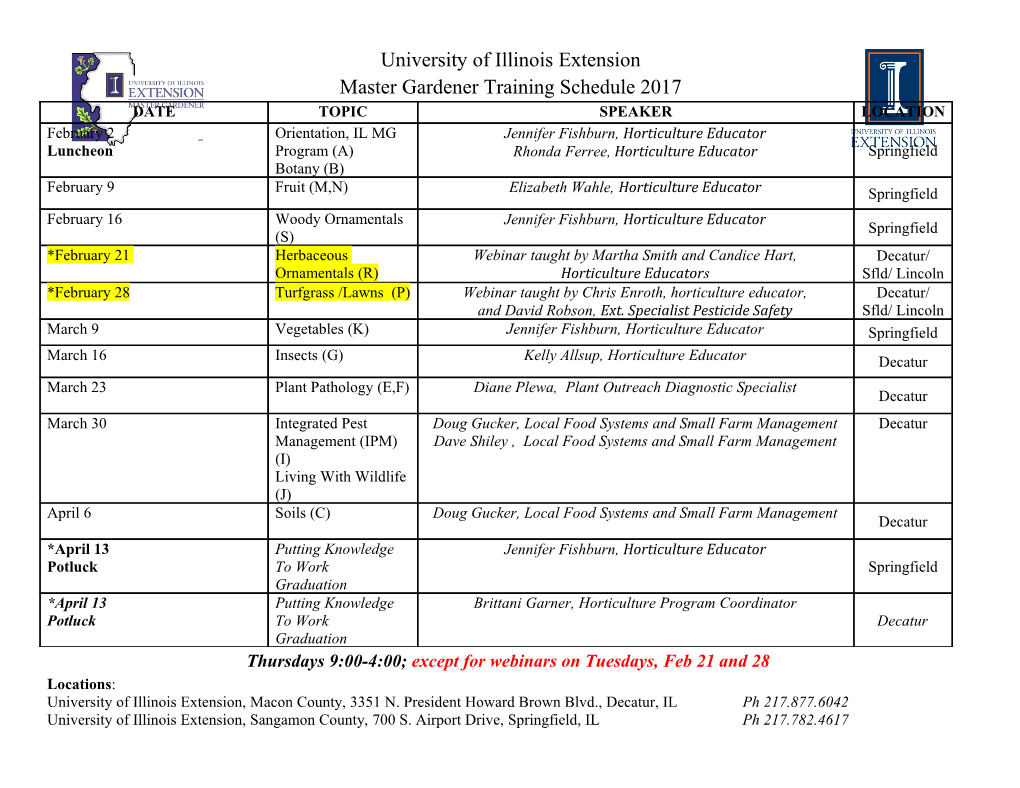
ICARUS 40, 49--59 (1979) Zodiacal Light Surface Brightness Measurements by Atmosphere Explorer-C MARSHA R. TORR*, D. G. TORR*, AND R. STENCEL~ Space Physics Research Laboratory, University of Michigan, Ann Arbor, Michigan 48109 Received October 13, 1977; revised February 13, 1979 Using the visible airglow photometer on the Atmosphere Explorer-C satellite, we have mapped the zodiacal light surface brightness at the wavelengths monitored by the instrument: 3371, 4278, 5200, 5577, 6300, and 7319 .~. The study constitutes a survey over this wavelength range, covering most of the celestial sphere, from altitudes above the atmospheric emissions, and free from atmo- spheric scattering and attenuation. The intensity variations reveal enhancements near elongations of 130°, and possibly near 60°, at all wavelengths. The intensity of the zodiacal light near the ecliptic pole is found to be -30 S,0. The color ratio with respect to the Sun is found to be redder than the Sun (0.7) at all elongations. 1. INTRODUCTION as a function of ecliptic latitude (/3) and Much of our knowledge of dust and par- heliocentric ecliptic longitude (~) for 3371, ticulate matter in the solar system comes 4278, 5200, 5577, 6300, and 7319 ~. The from observations of sunlight scattered off results are compared with a Mie-type scat- these particles. An addition to the data base tering model and with previous measure- of surface brightness measurements that ments. We determine color ratios, relative have been accumulated over the years is the to the Sun, for several pairs of wavelengths. large volume of measurements, made at 2. MEASUREMENTS high altitude and at several wavelengths, by The surface-brightness measurements the Visible Airglow Experiment (VAE) on used in this paper were made by the visible the Atmosphere Explorer satellites (Hays et airglow photometer on the AE-C satellite. al., 1973). This instrument has been described by Satellite measurements of the zodiacal Hays et al. (1973). The photometer contains light have an advantage over ground-based six interference filters, with bandwidths of measurements because of freedom from -20 A, mounted in a filter wheel so that any contamination by atmospheric emissions one of these can be moved into the optical and the problems of scattering and extinc- path. In addition, background and calibra- tion in the lower atmosphere. tion positions are available. The photometer The AE satellites have two modes of op- has two optical channels, oriented at right eration: despun, and spinning about an axis angles to the spin axis. The measurements normal to the orbital plane. This motion, discussed here were made with the wider of together with the precession of the orbital these channels, which had a half-angle field plane, allows almost complete coverage in of view of 3 ° . ecliptic latitude and longitude. In this paper The data base analyzed in what follows we present maps of the surface brightness was accumulated over a 10-month period between January and October 1974, during * Also affiliated with the National Institute for Tele- which the AE-C was in an elliptic orbit, and communications Research of the C.S.I.R., Johannes- burg, South Africa. measurements were made from altitudes t Present address: Goddard Space Flight Center, above the atmospheric emissions. In a Greenbelt, Md. 20771. separate paper (Torr et aL, 1977) we have 49 0019-1035/79/100049-11$02.00/0 Copyright © by Academic Press, Inc. All rights of reproduction in any form reserved. ~A Z [- Z < m 0 0 N © ~Z ,.-] > f~ ,.-]Z ~; : ~ :i ~ i ~/i/~ii!!~ i ¸I FIG. 1. Surface brightness maps on a polar coordinate system, as a function of ecliptic latitude and heliocentric ecliptic longitude. The units are R/,~. (a) 3371 /~; (b) 4278 ,~; (c) 5200 ,~; (d) 5577/~; (e) 6300 A; (f) 7319 A. 52 TORR, TORR, AND STENCEL addressed the question of stability of the galactic component has also been mapped sensitivity calibration of the instrument and will be reported separately.) over this time period. By monitoring an in- Thus sorted, the data have been averaged flight calibration source (as a check on the into 5 ° x 5 ° bins. The results are shown in stability of the photometer gain), and by Fig. 1 (a-f). We have assumed symmetry performing some coordinated measure- about the Sun in the ecliptic plane, and ments with ground-based stations, we are have averaged northern and southern eclip- confident that the sensitivity did not change tic latitudes, and east and west heliocentric significantly. longitudes. This is duscussed further below. Considerable care has been taken to Some gaps exist in the coverage. As a avoid measurements made under any condi- routine operational precaution, the photom- tions that might introduce spurious data. eter was not turned on when the orbital Some of these selection constraints have plane was within ~ 12° of the Sun. A result been discussed in a separate paper (Torr et of this was that no data were obtained al., 1977). We have not used measurements within 12° of the antisolar direction, pre- made below 500 km in altitude on the day cluding mapping of the gegenschein. The side, and below 450 km on the nightside. In orbital inclination of the AE-C satellite was addition, we have used only data measured 68.4 ° . This precluded observations being when the photometer was looking into the taken in the vicinity of the ecliptic pole. upward hemisphere, so as to avoid any Other unshaded areas of the maps were left slant observing paths downward through unfilled either because the sample was the atmosphere. The AE photometers have small (< 10) or the standard deviation was a two-stage baffle system, designed to at- large (>40%). Each 5 ° bin is therefore an tenuate scattered light from outside the field average of anywhere between 10 and sev- of view by 10 tz (Hays et al., 1963; Torr et eral hundred individual measurements. We al., 1977). This system makes it possible to hope to complete the maps at a later date by measure the zodiacal light at a surface combining information from the AE-D and brightness of-1 R/~ in the presence of AE-E satellites. sunlight (--5 × l0 H R//~ if viewed di- As the data have been carefully screened rectly). We have not used data taken closer to avoid atmospheric emissions and radia- than 35 ° to the Sun and 20 ° to the Moon. tion belt effects, the residual major non- We have avoided measurements made in zodiacal components are due to individual the vicinity of the South Atlantic Radiation stars and diffuse starlight. We consider the Anomaly by rejecting any data between effects of each of these in turn. For an in- geographic longitudes 100 ° W to 50°E, and strument operated from a platform that var- between geographic latitudes 15°N to 55°S. ies slowly with time, such as a ground- Outside this region, we have not used data based instrument, a first-magnitude star in a at altitudes above 1000 km, where fast 3 ° half-cone angle field of view would con- charged particles in the radiation belts tribute -134 SI0(V). A contribution of might affect the instrument (Torr et al., this magnitude would be comparable with 1977). the zodiacal component at large elongations The attitude of the satellite is known and thus would require removal for studies nominally to _+ l °. From this information we of these regions. However, the effect is have calculated the viewing direction of the much less severe for a spinning satellite- photometer in ecliptic coordinates and in borne instrument that progressively sweeps galactic coordinates. For the purposes of the celestial sphere. First, the spinning mo- the zodiacal maps, we have not used data tion of the vehicle effectively smears the within 30 ° of the galactic equator. (The field of view over the integration period, so ZODIACAL LIGHT MEASUREMENTS BY AE-C 53 that it is --54 square degrees, rather than 28 3.1. Variation in the Ecliptic Plane (for a 3 ° half-cone angle). The contribution The surface brightnesses shown in Fig. 1 of a first-magnitude star is thus ~<70 S10(V) are in units of rayleighs per angstrom, R//~ rather than 134 S10(V). A more significant (a unit commonly used in aeronomy, where point, however, is the fact that the star is 1 R = 106/4,n- photons cm-'-' sec-' sr-'). The only in the field of view for ~<0.13 sec, the most commonly used unit in zodiacal light duration of the integration period. The next work is $10, the number of tenth-magnitude time (days, weeks, or months later)that the stars per square degree. In Table I we show same 5 ° × 5 ° portion of the zodiacal light is a conversion between these two units at the scanned by the instrument, the stellar back- wavelengths considered in this paper. ground will be different. On the average Much of the earlier work has been re- 100 integration periods (accumulated over stricted to the plane of the ecliptic. Leinert the l0 months of measurement) comprise an (1975) has shown a comparison of intensity average surface brightness shown in a given measurements made by 16 workers since 5 ° x 5 ° bin at a particular wavelength. Thus 1964, as a function of elongation, ~' (where the contribution of the first-magnitude star ~' is equivalent to heliocentric longitude, E, is reduced to -0.7 S10(V) which corre- in the ecliptic plane; cos ~' = cos ~ cos/3). sponds to 3 x 10 -a R//~ at 5577 A and is not In Fig. 2 we show the variation of the AE significant. Stars of smaller magnitude will data for the six wavelengths analyzed here, not be detectable.
Details
-
File Typepdf
-
Upload Time-
-
Content LanguagesEnglish
-
Upload UserAnonymous/Not logged-in
-
File Pages11 Page
-
File Size-