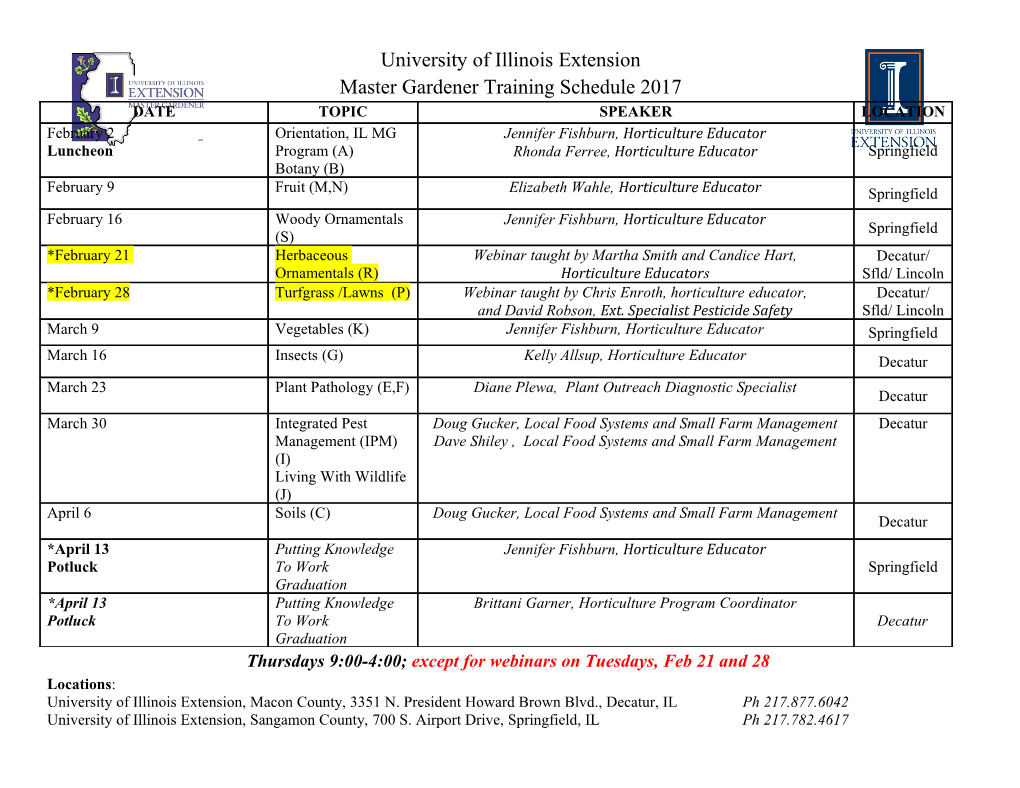
L A B O R A T O R I E S F O R T H E 21 ST C E N T U R Y : B EST P RACTICE G UIDE CH I L L E D BE A M S IN LABORATORIES : KE Y ST R A T E G I E S T O EN S U R E EF F E C T I V E DESIGN , CONSTRUCTION , A N D OPERATION • Overview describes how such beams work and Introduction their benefits in a laboratory setting, and presents three case studies. Laboratories commonly use far more energy than typi­ cal office buildings, primarily due to the intensive ventila­ • Designing Chilled Beam Systems discusses sizing tion required to address environmental, health, and safety a system, the controls and integration required, and concerns. As a result, facility designers and engineers are the challenges of modeling such systems. constantly seeking new ways to reduce energy consump­ • Construction examines system costs, how to hang tion while maintaining performance. Active chilled beam chilled beams, and code compliance. systems are gaining in popularity among laboratory designers because these systems allow ventilation require­ • Commissioning, Operations, and Maintenance ments to be decoupled from sensible heating and cooling summarizes how to commission, operate, and main­ loads. This decoupling eliminates the need for reheat tain chilled beam systems. coils for temperature control and reduces the fan energy • Appendix A contains a case study of the chilled required to maintain comfort. beam system installed at the Tahoe Center for Chilled beam systems are prevalent in European Environmental Sciences laboratory, which is also a commercial office buildings but have not yet been widely Labs21 partner project. applied in the U.S. Such systems offer many compelling benefits, including high cooling capacities, excellent This guide is one in a series created by the performance, and dramatic energy savings for little or no Laboratories for the 21st Century (Labs21) program, additional costs over conventional systems a joint program of the U.S. Environmental Protection Agency and U.S. Department of Energy (DOE). Geared The guide presents best practice strategies for design­ towards architects, engineers, and facilities managers, ing, constructing, operating, and maintaining chilled beam these guides provide information about technologies and systems in laboratories and is divided into the following practices to use in designing, constructing, and operating sections: safe, sustainable, high-performance laboratories. U.S. Department of Energy Energy Efficiency and Renewable Energy Federal Energy Management Program 2 LABS FOR THE 21ST CENTURY beam coils (see Figure 2). Passive beams are best suited to Overview applications with high heat loads and low ventilation air requirements, and therefore have limited application in How Chilled Beams Work most laboratories. This guide focuses only on active chilled beams, referred to from this point on simply as chilled Chilled beams (also called induction diffusers) are beams. fundamentally different from the all-air diffusers used throughout most U.S. buildings. There are two categories Chilled beams can accommodate sensible and latent of commonly used chilled beams: active and passive. loads. However, in properly designed laboratory environ­ Active chilled beams rely on air handlers supplying ments, chilled beams only provide the sensible cooling, outside air to condition a space and a cold water piping while the central air handling system provides the latent system that circulates water through integral cooling coils. cooling. This design avoids the additional costs of running The primary airflow from the air handling unit (AHU) to condensate drain piping to each beam in the building. the zone is introduced through small air jets, which typi­ When designing with chilled beams, there are two critical cally induce three to five times the amount of room air­ considerations: chilled water temperature and humidity flow through the beam’s coil (see Figure 1). The induction level in the conditioned space. If standard chilled water process provides local recirculation of room air. Passive (45°F) is used in the chilled beam, there is a risk of condens­ chilled beams rely simply on the natural convection in a ing water on the coil. To prevent such condensation, the room and have no direct air supply. As heat is transferred chilled beam water temperature must be actively main­ from the room air to the beam’s coil, the air is cooled and tained above the room air dew point. Both of these design falls into the occupied zone. As this occurs, warm room criteria are discussed in further detail under “System air up by the ceiling is drawn down through the passive Sizing” (in the “Designing Chilled Beam Systems” section). Figure 1. Active chilled beam systems use air supplied Figure 2. Passive chilled beam systems use the natural from an air handling unit. convection in a room and have no direct air supply. LABS FOR THE 21ST CENTURY 3 Benefits of Chilled Beams in Labs each room presents a huge potential energy loss. A chilled beam design avoids this energy loss by supplying a higher Chilled beams, while not appropriate for every labo­ temperature to each zone and dynamically cooling each ratory, can offer many benefits compared to the variable­ space individually. With a fixed amount of ventilation air, air-volume (VAV) reheat scheme commonly used in most chilled beams control the individual laboratory tempera­ standard lab systems (see Figure 3). In the VAV scheme, ture by adjusting the flow of chilled or hot water across boxes with reheat coils, control dampers, and airflow the beams to match any changing loads. In this case, using measurement devices are placed in each zone. While this chilled beams eliminates reheat energy and minimizes system meets building requirements, it uses significant outside air conditioning. amounts of fan and reheat energy. Case 2. Cooling-Load Driven Airflow The following typical laboratory cases demonstrate how chilled beams can reduce reheat energy, accurately When cooling loads in a lab drive the design airflow meet outside air requirements, and reduce building-wide rates, the use of chilled beams (which decouple the air and systems requirements, compared to VAV systems. The cooling requirements) can dramatically reduce the size of three cases differ in the amount of air required for ventila­ air systems. tion, safety, cooling, and fume hoods. In a typical VAV reheat system, each space meets its own cooling load by increasing the volume of cold air Case 1. Ventilation Driven Airflow supplied. This situation creates a dependent relationship All laboratories require a fixed amount of ventila­ between the airflow and the cooling capacity. In a chilled tion air to maintain safety. This case refers to laboratories beam system, cooling is accomplished with pumped where this “general exhaust” requirement (typically six chilled water rather than blown cold air. Water has a air changes per hour) drives the airflow, as distinct from a volumetric heat capacity 3,500 times that of air, which fume-hood–driven airflow covered in Case 3. translates to a reduction in fan energy by a factor of seven in typical pump and fan arrangements. On an annual Laboratories are dynamic buildings with a variety basis, the coil in the chilled beam accomplishes at least of rooms, each with their own general exhaust require­ half of the cooling with the remaining load handled by ments. In a typical laboratory HVAC (heating, ventilating, the primary air.1 Furthermore, the ramp-up of air typical and air conditioning) system with VAV reheat, the room in VAV reheat systems no longer occurs in labs with high with the highest heating load dictates the air temperature heat loads. In many detailed energy analyses of labs, cool­ supplied by the central AHU. Each lab space then reheats ing air and then reheating it can easily account for 20% of 1 the air, as needed. Reheating such high volumes of air for annual HVAC energy costs. Chilled Beam System VAV-Reheat System Reheat Energy To exhaust Reduced Duct Size To exhaust 6-20 ACH 6 ACH Flow Flow Flow Reheat Pressurization control control control Chilled control coil Ventilation Air Beams Ceiling Ventilation Air Ceiling Supplied at 55-70°F Supplied at 55°F 55-60°F supply air Decreased = 100% of lab Floor-to-Floor cooling Height Fume Fume hood in hood in Alcove Alcove Figure 3. Typical chilled beam and VAV reheat systems in labs. VAV reheat systems use significant amounts of fan and reheat energy compared to chilled beam systems. 4 LABS FOR THE 21ST CENTURY When chilled beam systems are used, ducting can be Step 1. Select a Beam Type downsized and the air handler central system reduced to Chilled beams vary in physical size, cooling capacity, handle less than half of the air needed by a typical system.1 airflow capacity, and many other parameters, depending The savings realized can be used to pay for the added pip­ on the manufacturer. For a given laboratory, the beam type ing and chilled beam capital costs. If modest reductions in selected typically depends on the following design param­ floor-to-floor height due to smaller ducting are taken into eters: maximum allowable design pressure drop for both account, using a chilled beam system can translate into air and water sides, chilled water supply temperature, sup­ an overall savings in construction costs and significantly ply air temperature, and allowable noise levels. reduced operation costs as well. Air and Water Pressure Drop Case 3. Fume Hood Driven Airflow Pressure drops across both the water side and air side The benefits of chilled beams are minimal for labs with of a chilled beam play a large role in specifying a system. a high density of fume hoods or other process exhaust.
Details
-
File Typepdf
-
Upload Time-
-
Content LanguagesEnglish
-
Upload UserAnonymous/Not logged-in
-
File Pages15 Page
-
File Size-