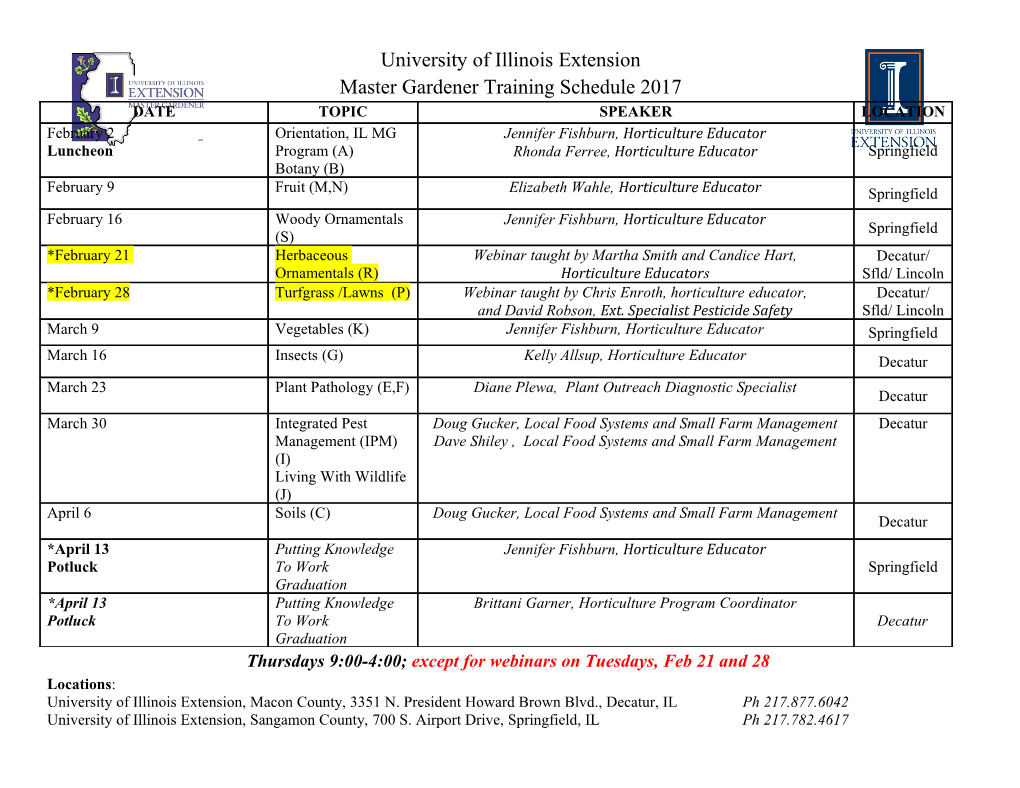
Su, Tina Yu-Ting (2016) The dehydrogenation reactions between LiH and organic amines for solid state hydrogen storage systems. PhD thesis. https://theses.gla.ac.uk/7863/ Copyright and moral rights for this work are retained by the author A copy can be downloaded for personal non-commercial research or study, without prior permission or charge This work cannot be reproduced or quoted extensively from without first obtaining permission in writing from the author The content must not be changed in any way or sold commercially in any format or medium without the formal permission of the author When referring to this work, full bibliographic details including the author, title, awarding institution and date of the thesis must be given Enlighten: Theses https://theses.gla.ac.uk/ [email protected] The Dehydrogenation Reactions between LiH and Organic Amines for Solid State Hydrogen Storage Systems Thesis submitted to the University of Glasgow for the Degree of Doctor of Philosophy Tina Yu-Ting Su School of Chemistry College of Science and Engineering University of Glasgow October 2016 Abstract Hydrogen is considered as an appealing alternative to fossil fuels in the pursuit of sustainable, secure and prosperous growth in the UK and abroad. However there exists a persisting bottleneck in the effective storage of hydrogen for mobile applications in order to facilitate a wide implementation of hydrogen fuel cells in the fossil fuel dependent transportation industry. To address this issue, new means of solid state chemical hydrogen storage are proposed in this thesis. This involves the coupling of LiH with three different organic amines: melamine, urea and dicyandiamide. In principle, thermodynamically favourable hydrogen release from these systems proceeds via the deprotonation of the protic N-H moieties by the hydridic metal hydride. Simultaneously hydrogen kinetics is expected to be enhanced over heavier hydrides by incorporating lithium ions in the proposed binary hydrogen storage systems. Whilst the concept has been successfully demonstrated by the results obtained in this work, it was observed that optimising the ball milling conditions is central in promoting hydrogen desorption in the proposed systems. The theoretical amount of 6.97 wt% by dry mass of hydrogen was released when heating a ball milled mixture of LiH and melamine (6:1 stoichiometry) to 320 °C. It was observed that ball milling introduces a disruption in the intermolecular hydrogen bonding network that exists in pristine melamine. This effect extends to a molecular level electron redistribution observed as a function of shifting IR bands. It was postulated that stable phases form during the first stages of dehydrogenation which contain the triazine skeleton. Dehydrogenation of this system yields a solid product Li 2NCN, which has been rehydrogenated back to melamine via hydrolysis under weak acidic conditions. On the other hand, the LiH and urea system (4:1 stoichiometry) desorbed approximately 5.8 wt% of hydrogen, from the theoretical capacity of 8.78 wt% (dry mass), by 270 °C accompanied by undesirable ammonia and trace amount of water release. The thermal dehydrogenation proceeds via the formation of Li(HN(CO)NH 2) at 104.5 °C; which then decomposes to LiOCN and unidentified phases containing C-N moieties by 230 °C. The final products are Li 2NCN and Li 2O (270 °C) with LiCN and Li 2CO 3 also detected under certain conditions. It was observed that ball milling can effectively supress ammonia formation. Furthermore results obtained from energetic ball milling experiments have indicated that the barrier to full dehydrogenation between LiH and urea is principally kinetic. Finally the dehydrogenation reaction between LiH and dicyandiamide system (4:1 stoichiometry) occurs through two distinct pathways dependent on the ball milling conditions. When ball milled at 450 RPM for 1 h, dehydrogenation proceeds alongside dicyandiamide condensation by 400 °C whilst at a slower milling speed of 400 RPM for 6h, decomposition occurs via a rapid gas desorption (H 2 i and NH 3) at 85 °C accompanied by sample foaming. The reactant dicyandiamide can be generated by hydrolysis using the product Li 2NCN. ii Table of contents Abstract i Table of contents iii List of figures and schematics v List of tables x Acknowledgement xi 1.0 Introduction 1 1.1 Properties of LiH 5 1.2 Li-N-H systems 6 1.2.1 Properties of Li-N-H materials 7 1.2.2 Ionic diffusion and Frenkel pair formation in LiH and Li-N-H materials 10 1.2.3 Thermolysis and cycling of Li-N-H materials 10 1.2.4 Proposed reaction mechanisms 12 1.2.4.1 Direct acid-base mechanism 12 1.2.4.2 Ammonia mediated mechanism 13 1.2.5 Means to enhance dehydrogenation between LiH and LiNH 2 15 1.2.5.1 Ti catalysts 15 1.2.5.2 Other catalysts 15 1.2.5.3 Ball milling 16 1.2.5.4 Confinement 16 1.3 LiH·B-N compounds 17 1.3.1 LiH·AB 17 1.3.1.1 Thermolysis of AB 18 1.3.1.2 Lithium amidoborane 18 1.3.1.3 The formation of LiAB 19 1.3.1.4 Thermolysis of LiAB 20 1.3.1.5 Dehydrogenation mechanism of LiAB 21 1.3.1.6 LiAB regeneration 22 1.3.2 LiH·HB 23 1.3.2.1 Thermolysis of HB 24 1.3.2.2 Formation of lithium hydrazine borane 24 1.3.2.3 Dehydrogenation of α-LiHB 25 1.2.3.4 The formation of β-LiHB 26 1.4 Summary and project objectives 25 1.5 References 28 2.0 Experimental 32 2.1 Sample handling 32 iii 2.2 Sample preparation 33 2.2.1 Planetary ball milling 33 2.2.2 Pressure monitored ball milling 34 2.2.3 Furnaces 34 2.3 Analysis and characterisation techniques 35 2.3.1 Crystallography and diffraction 35 2.3.1.1 Powder X-ray Diffraction 38 2.3.1.2 Powder Neutron Diffraction 42 2.3.1.3 Rietveld Refinement 44 2.3.2 Other spectroscopy techniques 47 2.3.2.1 IR spectroscopy 47 2.3.2.2 Solid state NMR 49 2.3.3 Scanning Electron Microscopy 52 2.3.4 Thermal Analysis 54 2.3.4.1 Data collection 55 2.3.4.2 Mass Spectrometry 56 2.4 References 58 3.0 Dehydrogenation reaction between LiH and melamine 59 3.1 Introduction 59 3.1.1 Properties of melamine 59 3.1.2 Thermolysis of melamine 61 3.1.2 Melamine in hydrogen storage 63 3.2 Experimental 63 3.2.1 Materials 63 3.2.2 Sample preparation 64 3.2.2.1 Ball milling 64 3.2.3 Characterisation techniques 65 3.2.3.1 Powder Neutron Diffraction 65 3.2.3.2 Solid state NMR 65 3.2.3.2.1 CuCl 2 doping 65 3.2.3.2.2 2H and 15 N MAS NMR measurements 65 3.3 Dehydrogenation mechanism between LiH and melamine 66 3.3.1 System reproducibility 66 3.3.2 Powder neutron experiments 73 3.3.3 Solid state NMR experiments 76 3.3.4 Isotopic labelling experiments 81 3.4 Discussion 89 3.4.1 Structural changes to facilitate hydrogen desorption 89 3.4.2 Dehydrogenation scheme 90 iv 3.5 Regeneration of Li 2NCN 92 3.6 Conclusions and outlook 95 3.7 References 96 4.0 Dehydrogenation reaction between LiH and urea 98 4.1 Introduction 98 4.1.1 The structure of urea 99 4.1.2 Urea chemistry 101 4.1.3 Decomposition of urea 103 4.1.3.1 Hydrolysis 103 4.1.3.2 Thermolysis 103 4.1.4 Urea as a hydrogen source 105 4.2 Experimental 107 4.2.1 Materials 107 4.2.2 Ball milling 107 4.2.3 Characterisation techniques 108 4.3 Results and discussion 109 4.3.1 Intimately mixed 4 LiH : urea 109 4.3.1.1 Region 1 – RT to 140 °C 110 4.3.1.2 Region 2 – 160 to 230 °C 114 4.3.1.3 Region 3 – 230 to 300 °C 119 4.3.2 Reaction stoichiometry studies 120 4.3.2.1 Sample U-04, 1LiH:urea 123 4.3.2.2 LiH : urea ratio of 2:1, 3:1 and 4:1 125 4.3.3 Ball milling experiments 128 4.3.3.1 Effect of increasing ball milling speed 129 4.3.3.2 Effects of ball milling on reactant activity 132 4.3.3.3 Activation energy calculation 137 4.3.3.4 Summary 140 4.4 The efficacy of the LiH:urea hydrogen storage system 141 4.5 Conclusions and future work 142 4.6 References 143 5.0 Dehydrogenation between LiH and dicyandiamide 146 5.1 Introduction 146 5.1.1 Properties of dicyandiamide 146 5.1.2 Guanidine in hydrogen storage 149 5.2 Experimental 150 5.3 Results and discussion 150 5.4 Conclusion 167 v 5.5 References 165 6.0 Concluding remarks 169 Appendices 171 Appendix A 171 Appendix B 172 Appendix C 173 Appendix D 177 Appendix E 178 Appendix F 180 Appendix G 182 vi List of Figures and Schematics Figure 1-1 Total energy consumption in Europe between 1990 and 2013, by fuel type 1 Figure 1-2 2015-2016 UK energy consumption by sector 2 Figure 1-3 Schematic of a PEMFC for automobiles 3 Figure 1-4 Unit cell of LiH 5 Figure 1-5 Unit cells of (a) α-Li 3N and (b) β-Li 3N 8 Unit cells of Li 2NH without hydrogen positions (a) Fm 3m, (b) F43m and (c) Figure 1-6 9 LiNH 2 Figure 1-7 Li-N-H ternary phase diagram by CALPHAD simulations 11 Figure 1-8 Simplified schematic of the direct redox dehydrogenation mechanism 12 Simplified schematic of the ammonia mediated dehydrogenation Figure 1-9 13 mechanism Figure 1-10 TEM images of LiTi 2O4 post hydrogenation 15 Figure 1-11 Unit cell of NH 3BH 3 18 Figure 1-12 Unit cell of LiNH 2BH 3 19 Figure 1-13 Unit cell of N2H4BH 3 23 Figure 1-14 Unit cells of α-LiN 2H3BH 3 25 Figure 1-15 Compounds identified as dehydrogenation pairs with LiH 26 Figure 2-1 Air sensitive clamp and the 50 ml stainless steel milling jars 33 Figure 2-2 The Retsch PM 100 planetary mill with the spider clamp 34 Figure 2-3 A crystal unit cell 35 Figure 2-4 Diffraction of a radiation by a crystal 36 Figure 2-5 Debye-Scherrer cone diffraction from a powder sample 37 Figure 2-6 X-ray interaction with matter 39 Figure 2-7 The Bruker D8 powder diffractometer set up 39 The flat plate (reflection, left) and capillary (transmission, right) sample Figure 2-8 40 geometries Figure 2-9 Silica capillary alignment set
Details
-
File Typepdf
-
Upload Time-
-
Content LanguagesEnglish
-
Upload UserAnonymous/Not logged-in
-
File Pages198 Page
-
File Size-