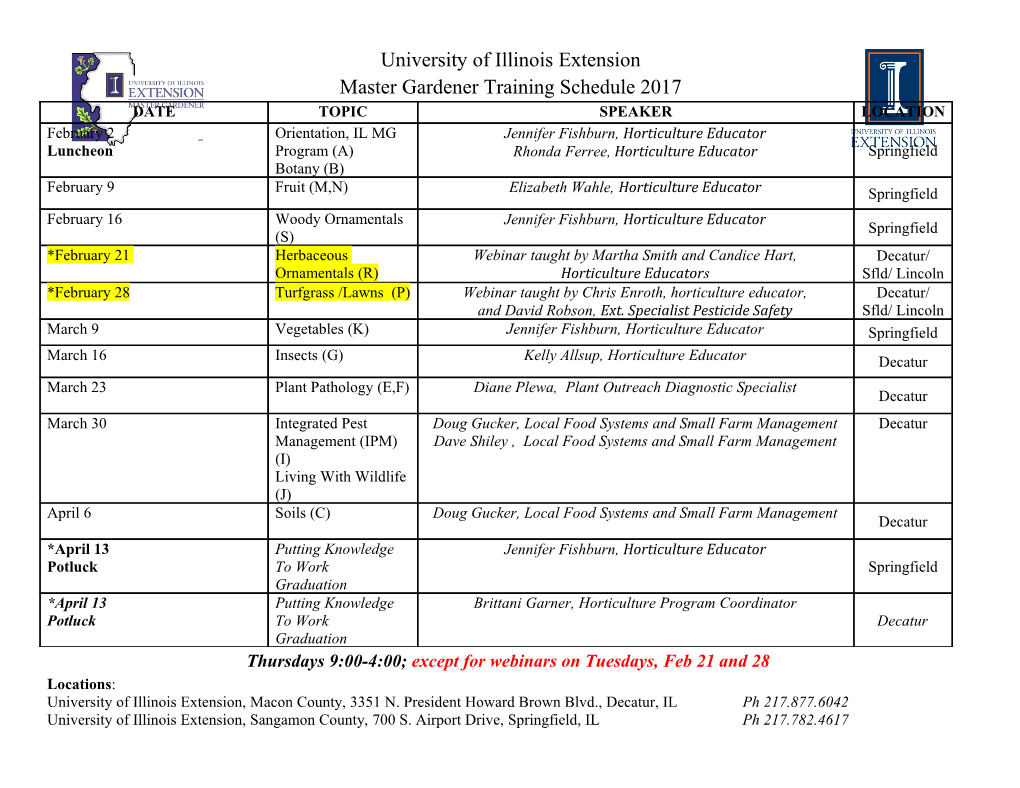
The Astrophysical Journal Supplement Series, 241:36 (32pp), 2019 April https://doi.org/10.3847/1538-4365/ab0ca4 © 2019. The American Astronomical Society. Chandra Early-type Galaxy Atlas Dong-Woo Kim1 , Craig Anderson1, Douglas Burke1 , Raffaele D’Abrusco1 , Giuseppina Fabbiano1 , Antonella Fruscione1, Jennifer Lauer1, Michael McCollough1 , Douglas Morgan1, Amy Mossman1, Ewan O’Sullivan1 , Alessandro Paggi1 , Saeqa Vrtilek1, and Ginevra Trinchieri2 1 Center for Astrophysics|Harvard & Smithsonian 60 Garden Street, Cambridge, MA 02138, USA 2 INAF-Osservatorio Astronomico di Brera, Via Brera 28, I-20121 Milan, Italy Received 2018 November 30; revised 2019 February 25; accepted 2019 March 3; published 2019 April 16 Abstract The hot interstellar medium (ISM) in early-type galaxies (ETGs) plays a crucial role in understanding their formation and evolution. The structural features of the hot gas identified by Chandra observations point to key evolutionary mechanisms, (e.g., active galactic nuclei, AGN, and stellar feedback, merging history). In our Chandra Galaxy Atlas (CGA) project, taking full advantage of the Chandra capabilities, we systematically analyzed the archival Chandra data of 70 ETGs and produced uniform data products for the hot gas properties. The primary data products are spatially resolved 2D spectral maps of the hot gas from individual galaxies. We emphasize that new features can be identified in the spectral maps that are not readily visible in the surface brightness maps. The high-level images can be viewed at the dedicated CGA website, and the CGA data products can be downloaded to compare with data at other wavelengths and to perform further analyses. Using our data products, we address a few focused science topics. Key words: galaxies: elliptical and lenticular, cD – X-rays: galaxies 1. Introduction have often focused on the global properties or 1D radial fi ( ) For the past two decades, the Chandra X-ray Observatory pro les e.g., Kim & Fabbiano 2015; Lakhchaura et al. 2018 . has revolutionized many essential science subjects in astron- To explore the 2D distribution of spectral properties of the hot gas, we applied four different spatial binning techniques. In omy and astrophysics. The main driving force is its ( unprecedentedly high spatial resolution3—the capability to particular, the 2D spectral maps of the hot gas temperature, fi normalized emission measure, projected pseudo-pressure, and resolve ne structures in a subarcsecond spatial scale. The ) study of the hot interstellar medium (ISM) in early-type projected pseudo-entropy can reveal unique features that may fi galaxies (ETGs) can be taken to a new level thanks to this not be visible in 1D radial pro les or the 2D surface resolution. The hot ISM, which is the dominant phase in ETGs brightness maps alone. (e.g., see Kim & Pellegrini 2012), plays a crucial role in Although many galaxies were previously investigated, those understanding the formation and evolution of the host galaxies. studies were mostly done individually (see Appendix B).We Various structural features of the hot ISM, which was provide uniformly reduced data products so that the entire previously considered as smooth and featureless, have been sample of ETGs can be examined consistently. We also identified by Chandra. They include cavities, cold fronts, note that some Chandra data are extensively investigated for filaments, and tails, which are closely related to critical the first time as part of this project (e.g., NGC 1132 by Kim astrophysical mechanisms for the galaxy formation and et al. 2018). We make use of new and improved data analysis evolution, e.g., AGN feedback, merging history, accretion, (and statistical) techniques (e.g., CIAO4 s/w package v4.9), stripping, and star formation (SF) and its quenching (e.g., see instrument calibration data (in Chandra CALDB5 v4.7), and Kim & Pellegrini 2012 and references therein). atomic data (in ATOMDB6 v3). Using the first four years of Chandra observations, Diehl & This paper is organized as follows. In Section 2 we describe Statler (2007, 2008a, 2008b) assembled a sample of 54 nearby the sample selection and observational information of each (D100 Mpc) ETGs (or 36 with temperature profiles). Chandra archival data. In Section 3 we describe the Chandra These authors produced a systematic characterization of the data analysis techniques and related issues. In Section 4 we hot gas morphology and compared this morphology with the present the data products of the Chandra Galaxy Atlas (CGA), optical stellar distribution, radio emission, and AGN proper- our guidelines for general users on how to use the CGA data, ties. Using the first 15 yr of Chandra observations, we have and data caveats in the current version. In Section 5 we explain expanded the nearby ETG sample to 70 galaxies and current and future focused science goals. In Appendix A we list produced spatially resolved homogeneous data products with the CGA data products (downloadable) and their descriptions additional spectral information. Previous archival studies in detail. In Appendix B we present the notes on individual galaxies. Throughout this paper, we quote errors at the 1σ 3 http://cxc.harvard.edu/cdo/about_chandra/overview_cxo.html significance level. Original content from this work may be used under the terms 4 // / / of the Creative Commons Attribution 3.0 licence. Any further http: cxc.harvard.edu ciao 5 distribution of this work must maintain attribution to the author(s) and the title http://cxc.harvard.edu/caldb/ of the work, journal citation and DOI. 6 http://www.atomdb.org 1 The Astrophysical Journal Supplement Series, 241:36 (32pp), 2019 April Kim et al. 2. Sample Selection and Chandra Observations For ACIS-I observations, we use the four front-illuminated ( < chips I0-I3 (CCDID=0–3), while for ACIS-S, we use the Our sample contains 70 E and S0 galaxies type 0, based ( = ) on RC37) for which observations are available from the public back-illuminated chip S3 CCDID 7 , where the target lies, ( = ) Chandra archive (up to AO15). Because many giant galaxies and the front-illuminated chip S2 CCDID 6 , where the / extended diffuse emission is often visible. Because the point- are in galaxy groups clusters in the RC3 catalog, our sample 10 includes several examples of brightest group/cluster galaxies spread function (PSF) becomes large at large OAAs, the other (BCGs). However, we exclude large groups/clusters by chips are generally not useful for our purposes. However, for a ( limiting TGAS below ∼1.5 keV, because TGAS is a good few special cases, we use a nonstandard set of chips see measure of the total mass of the system. About 80% of the Table 2). Similarly, we do not use observations where the target ( > ) sample galaxies have TGAS=0.3–1.0 keV. galaxy is at a large OAA typically OAA 4 arcmin because We use both ACIS-I and ACIS-S Chandra observations,8 of the large PSF. but exclude observations with grating. We limit the minimum Figure 1(a) illustrates an example of merging six (two ACIS-I exposure time to 10 ks to exclude snapshot observations for and four ACIS-S) Chandra observations of NGC 1399. AGN studies. In Table 1 we list our sample with the basic Figure 1(b) shows the DSS11 optical image of the same region galaxy information (e.g., R.A., decl., distance, size, and K-band of the sky. In all observations, the center of NGC 1399 is luminosity) and the total Chandra exposure time (see the within 1.5 arcmin from the aim point. footnotes below). Figure 2 shows the entire data processing workflow. Once As multiple Chandra observations are often merged for a ACIS data are downloaded from the Chandra archive, we run given galaxy, we assign a unique merge id, or mid in short the ACIS level 2 processing using the CIAO tool chandra_r- (Column 12 in Table 1; Column 3 in Table 2) to each galaxy epro.12 This step ensures that the most recent calibration data data set. If only one Chandra observation is used, the mid is the (e.g., CTI correction, time-dependent ACIS gain, bad pixels) same as the Chandra observation ID (obsid). are applied. To correct the relative positional error among For multiple observations, the mid consists of five digits, like multiple observations, we detect point sources from individual an obsid. The first digit is 9, followed by two digits indicating observations using the CIAO tool wavdetect (with scale=1, 2, the number of obsids used. The last two digits (usually “01”) 4, 8 and sigthresh=10−6) in a C-band image (0.5–5 keV, see are unique serial numbers to separate different combinations of Table 3 for the definitions of energy bands used in CGA) made observation parameters (e.g., set of obsids and set of chips). with the CIAO tool fluximage. Note that we run fluximage The exposure time of this merged data set (Column 13 in again after removing point sources detected on the merged data Table 1) is determined by summing the LIVETIME of the chip in Section 3.2. Then, we register bright (net counts>30) point where the galaxy center lies, after removing background flares sources commonly detected in a pair of observations, using the that were determined for each chip of each obsid. See Section 3 CIAO tool reproject_aspect (with radius=2 and resi- for details on how we removed background flares. dlim=1). This positional correction is usually small (ΔR.A. In Table 2 we list the Chandra observational information for and Δdecl.<0 5) but necessary for the Chandra observations each obsid, grouped by galaxy. They include the observation with a high spatial resolution. We rerun chandra_repro for the date (Column 4), off-axis angle (OAA; in arcmininutes) of the second time to apply the newly determined aspect solutions.
Details
-
File Typepdf
-
Upload Time-
-
Content LanguagesEnglish
-
Upload UserAnonymous/Not logged-in
-
File Pages32 Page
-
File Size-