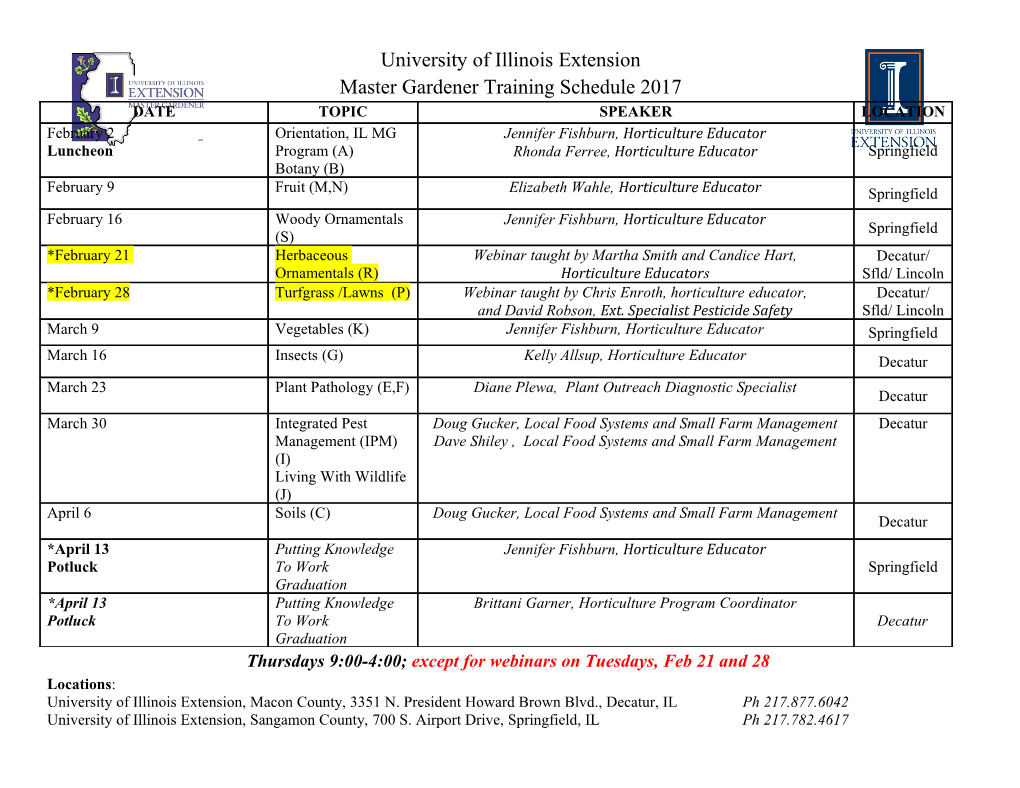
Downloaded from http://sp.lyellcollection.org/ by guest on September 30, 2021 Salt tectonics: some aspects of deformation mechanics IAN DAVISON, IAN ALSOP & DEREK BLUNDELL Department of Geology, Royal Holloway, University of London, Egham, TW20 OEX, UK This volume is dedicated to studies of the defor- Deformation mechanisms mation of evaporite rocks in pillows and diapirs, and the surrounding sedimentary overburden rocks Burliga, Davison et al., Sans et al., Smith and and sediments. Salt diapirs have become the focus Talbot & Alavi provide new insights into the in- of attention in the last forty years, because of their ternal deformation patterns in salt from mesoscopic observations in deformed bodies. Shear zones are strategic importance in controlling hydrocarbon commonly developed parallel to bedding in potassic reserves, and their unique physical properties enable storage of hydrocarbons and toxic waste. horizons (Sans et al.), where the salt becomes Their economic importance is unique on the Earth's gneissose with X:Z axial ratios of crystals reaching commonly around 4:1 in Zechstein salt (Smith), surface, as evaporites in the Middle East are and Red Sea diapirs (Davison et al., Fig. 1). responsible for trapping up to 60% of its hydro- carbon reserves (Edgell). Relatively undeformed halite layers are carried laterally (Smith) and upwards as rafts between Salt also produces some of the most complex and beautiful deformation features on the Earth's shear zones into diapirs (Bnrliga), and undeformed surface, although few of these surface exposures halite rafts are often transported in a highly-sheared have been examined in detail. The first section of sylvinite matrix (Sans et al.). this volume consists of analyses of outcrop, cave, Average halite grain sizes generally range mine and borehole information which add to our between 5-10 mm in North Sea Zechstein halite (our own observations) and 15-20 mm in deformed general understanding of the internal diapir defor- mation patterns and overburden tectonics. This is Gulf Coast Louann Salt (Talbot & Jackson 1987). At this size, both pressure solution and dislocation followed by papers mainly based on seismic reflec- tion profile interpretation, which provide accurate creep will be active mechanisms of deformation (Van Keken et al. 1993) and characteristics of both documentation of salt tectonics in the NW Europe Zechstein Basin (Buchanan et ai., Stewart et ai., creep mechanisms have been described in natural salt (Spiers et al. 1990). Zirngast), Persian Gulf (Edgell), and the Angolan Margin (Spathopoulos). Salt can fault in a brittle mode at high strain rates Salt diapirism is principally controlled by the (Fig. 2, Smith; Davison et al.). Long range theology of the overburden rocks and physical methane gas migration occurs through fractures in salt in the Algarve Basin, Portugal (Terrinha et al. models have given us important new insights into the deformation of overburden. Deformation ex- 1994) and in the Sergipe-Alagoas Basin, NE Brazil (Fig. 2). This has important implications for both periments are presented on overburden around salt structures (Alsop), salt sheet segmentation (Koyi), hydrocarbon potential and toxic waste disposal. and development of giant counter-regional faults on the Brazilian continental margin (Szatmari et ai.). Petersen & Lerche investigate the influence of salt Flow patterns inside the source layer sheets and diapirs on thermal history. Salt has a Fold wavelengths of salt layers that have original thermal conductivity (K) which is up to three times thicknesses greater than 1 km with one kilometre of greater than the surrounding sediments, but K overburden will typically be about 20-30 km in the decreases by up to a third downwards through a 5 North Sea (Fig. 3). The initial wavelength of the km high salt diapir. In contrast, increasing com- perturbations controls the maximum amount of salt paction produces higher K in the sedimentary rocks that is available to flow into an individual salt struc- with increasing depth. Variable conductivity models ture. Assuming a circular drainage radius of 10 km with compaction and temperature indicate that for North Sea examples implies that for a 1.5 km thermal effects are much larger at the top of the salt thick salt layer approximately 470 km 3 of salt could structure than at the base. be transported into a single diapir. This is much The following sections summarize some of the greater than the amount of salt contained in most fundamental aspects relating to salt tectonics North Sea diapirs which will be around 25-75 km 3. covered by this thematic set of papers, but it is not The rate of radial flow of this amount of salt will intended to be a full review. increase as the diapir is approached, so that there is a mass acceleration and constriction of flow From ALSOP, G. I., BLUNDELL,D. J. & DAVlSON,I. (eds), 1996, Salt Tectonics, Geological Society Special Publication No. 100, pp. 1-10. Downloaded from http://sp.lyellcollection.org/ by guest on September 30, 2021 2 I. DAVISON ETAL. Fig. 1. Photograph of halite fabric in the centre of a mature Red Sea diapir in NW Yemen (Davison et al.). This coarse grain size (5-10 mm) is typical of many major diapir provinces and suggests that diapirs deform by both pressure solution and dislocation creep. Note the vertical extension lineation in the salt produced by vertical flow in the diapir neck. Downloaded from http://sp.lyellcollection.org/ by guest on September 30, 2021 SALT TECTONICS 3 Fig. 2. Fault in Aptian age halite and sylvinite from the PETROMISA Mine near Aracaju, Sergipe-Alagoas Basin, NE Brazil. The salt lies at a present depth of approximately 300 m with overburden of Cretacous age. Coarse-grained halite has recrystallized along the fault plane. Open fractures filled with methane are also present in this mine. towards the diapir (Fig. 4). Strain rates in the diapir apparently undeformed surfaces in diapiric heads neck could be up to 80 times larger than the average (Figs 4 & 5). flow rate in the source layer (our own calculations Flow of viscous salt in a confined layer exhibits a from experimantal models using SGM polymer, Poiseuille velocity profile, with the highest flow Fig. 4). Vertical flow in the centre of the diapiric velocity in the centre of the layer (Fig. 6a). neck produces extreme prolate strains (Davison et Intervening sedimentary layers within the evaporite al.). These are nullified at the top of the diapir sequence produce a relative decrease in the rate of where vertical flattening takes place; this may leave lateral flow due to increased drag effects (Fig. 6d). Downloaded from http://sp.lyellcollection.org/ by guest on September 30, 2021 4 I. DAVISON ETAL. T r 1 a) I I~ ~ 20E 3*E " i!ii '::: # 9 9 . ""t~7'/= 9 9 . 9. 9 . ~ 9 9 ,Ill O 9 9 ii 9 9 9J, =, " *o 9 9 9 9 el 9 9 9 et Holland; = it "~ " " 9 9 ~ ' # t i 9 : I 9 9 I I 9 t 9 %,. * 9 t 9 ,' pe ~" 9 B t Germany 9 " . 9 * e @-- Specino sl,ose o+ ;":" . .~ , Sa,tp+oemeot " '1." : .- structures " ~ o531 ~Spacing ellipse ~ % o ~, ~ 50km = 9 ~ 9 I ~ Salt diapir ~~i~ L ~ salt pillow 9b) ~ 9 4 "', 50kin Q 9 9 "'.Spacing ellipse ID Salt piercements gO0 9 9 ! ~==:Z~) Salt pillows /v',, ,. and ridges 9 ~ Limit of "..l 9 8 9 9 ~echsteein Sal't .'..~... : ....... -- ~ .... 2, 57 ~ 30'N . 9 - 9 9 9e'qh 9 9 ~ 9 O ~ Q Q 9 9 o:| 9 e 9 ~ +&E Z 9~7 " ~ Fig. 3. Summaryof salt structures in various provinces of the North Sea Zechstein Basin: (a) southern North Sea (Hughes & Davison 1993). Fry plots indicate average minimum spacing between structures in a NE-SW direction is 25 km, and 58 km in a NW-SE direction. (b) Norwegian-Danish basin (Hospers et al. 1988). Average spacing between structures is 23 km in a NE-SW direction, and 39 km in an NW- SE direction. (c) Northern Germany (Trusheim 1960). Average spacing between structures is 31 km in an E-W direction and 26 km in a N-S direction. Each layer behaves individually when controlling may have a viscosity around 1018 Pa s (Van Keken lateral flow, but the layers must act together during et al. 1993). It is difficult to make direct estimates vertical flow perpendicular to the initially horizon- of large-scale effective viscosities of diapirs over tal layering (Fig. 6e). Couette plate type flow (Fig. long time periods. Different parts of the diapir 6b) will occur in the source layer where overburden deform at different velocities and by different immediately above is shortening or extending. mechanisms (Van Keken et al. 1993). Analogies Hence, salt at the top of the layer is dragged by the with physical or mathematical models give esti- overburden and appreciable mass movement may mates of effective viscosities of diapir source layers occur, with salt being dragged along detachments to which vary between 1016 and 1018 Pa s (e.g. Koyi thicken downslope (Fig. 6f). Downslope thickening 1988, 1991; Nalpas & Brun 1993). However, the of salt on the western and eastern platforms of the viscosity of the salt has little to do with the trigger- Central Graben may have been produced by flow of ing of diapirism; which is primarily dependent on salt into the Graben despite the natural buoyancy of the strength and stress state of the overburden. The the salt. few examples of viscosity contrast estimates of salt and overburden in natural examples have been based on initial wavelength-thickness estimates Effective viscosity of salt and overburden using Ramberg theory (Rrnnlund 1989; Hughes & Davison 1993). These estimates give sedimentary and anisotropy rock:evaporite rock viscosity ratios of 50-104.
Details
-
File Typepdf
-
Upload Time-
-
Content LanguagesEnglish
-
Upload UserAnonymous/Not logged-in
-
File Pages10 Page
-
File Size-