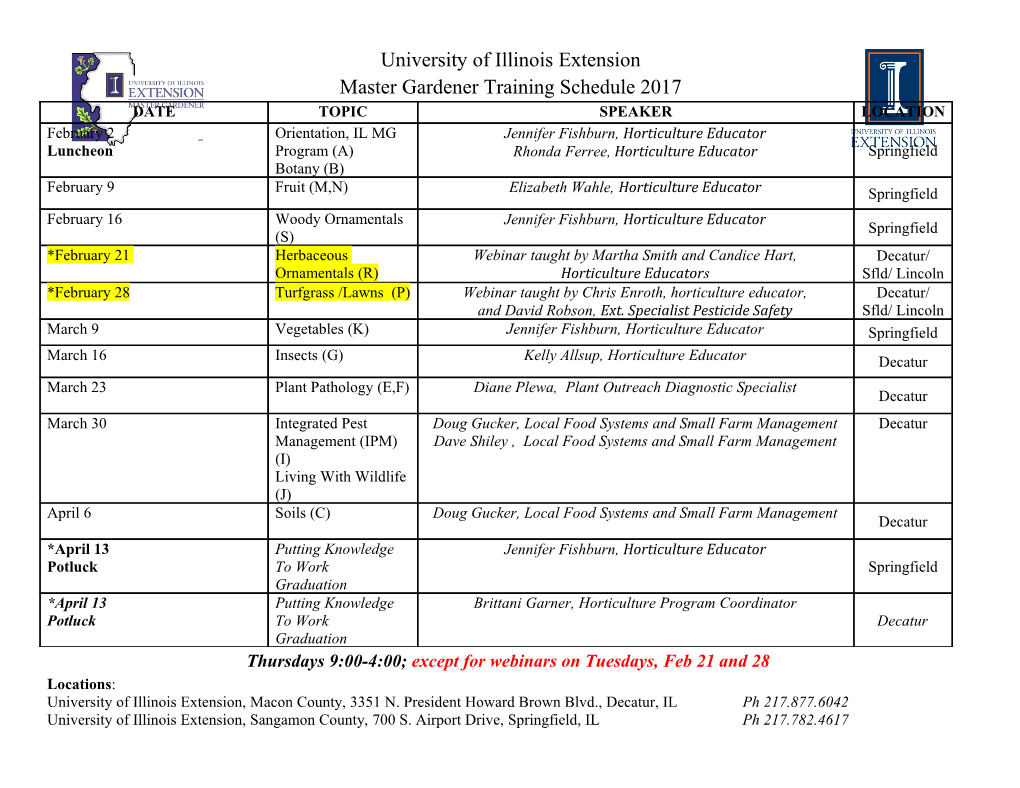
bioRxiv preprint doi: https://doi.org/10.1101/540435; this version posted February 5, 2019. The copyright holder for this preprint (which was not certified by peer review) is the author/funder, who has granted bioRxiv a license to display the preprint in perpetuity. It is made available under aCC-BY-NC-ND 4.0 International license. 1 Sulfur-oxidizing symbionts without canonical genes 2 for autotrophic CO2 fixation 3 Classification: Biological Sciences – Environmental Sciences 4 Brandon K. B. Seah*1,7, Chakkiath Paul Antony1,8, Bruno Huettel2, Jan Zarzycki3, 5 Lennart Schada von Borzyskowski3, Tobias J. Erb3, Angela Kouris4, Manuel Kleiner5, 6 Manuel Liebeke1, Nicole Dubilier1,6, Harald R. Gruber-Vodicka1 7 1 Max Planck Institute for Marine Microbiology, Celsiusstraße 1, 28359 Bremen, Germany 8 2 Max Planck Genome Centre Cologne, Max Planck Institute for Plant Breeding Research, Carl-von-Linné-Weg 9 10, 50829 Cologne, Germany 10 3 Max Planck Institute for Terrestrial Microbiology, Karl-von-Frisch-Str. 10, 35043 Marburg, Germany 11 4 Energy Bioengineering and Geomicrobiology Group, University of Calgary, 2500 University Drive Northwest, 12 Calgary, Alberta T2N 1N4, Canada 13 5 Department of Plant and Microbial Biology, North Carolina State University, Raleigh 27695, North Carolina, 14 United States of America 15 6 MARUM, Center for Marine Environmental Sciences, University of Bremen, 28359 Bremen, Germany 16 7 Current address: Max Planck Institute for Developmental Biology, Max-Planck-Ring 5, 72076 Tübingen, 17 Germany 18 8 Current address: Red Sea Research Center, Biological and Environmental Sciences and Engineering (BESE) 19 Division, King Abdullah University of Science and Technology (KAUST), Thuwal 23955, Kingdom of Saudi 20 Arabia 21 * Corresponding author: Brandon K. B. Seah, Max Planck Institute for Developmental Biology, Max-Planck- 22 Ring 5, 72076 Tübingen, Germany; tel. +49 7071 601 1342; [email protected] / 23 [email protected] 1 bioRxiv preprint doi: https://doi.org/10.1101/540435; this version posted February 5, 2019. The copyright holder for this preprint (which was not certified by peer review) is the author/funder, who has granted bioRxiv a license to display the preprint in perpetuity. It is made available under aCC-BY-NC-ND 4.0 International license. 24 Abstract 25 Since the discovery of symbioses between sulfur-oxidizing (thiotrophic) bacteria and 26 invertebrates at hydrothermal vents over 40 years ago, it has been assumed that autotrophic 27 fixation of CO2 by the symbionts drives these nutritional associations. In this study, we 28 investigated Candidatus Kentron, the clade of symbionts hosted by Kentrophoros, a diverse 29 genus of ciliates which are found in marine coastal sediments around the world. Despite 30 being the main food source for their hosts, Kentron lack the key canonical genes for any of 31 the known pathways for autotrophic fixation, and have a carbon stable isotope fingerprint 32 unlike other thiotrophic symbionts from similar habitats. Our genomic and transcriptomic 33 analyses instead found metabolic features consistent with growth on organic carbon, 34 especially organic and amino acids, for which they have abundant uptake transporters. All 35 known thiotrophic symbionts have converged on using reduced sulfur to generate energy 36 lithotrophically, but they are diverse in their carbon sources. Some clades are obligate 37 autotrophs, while many are mixotrophs that can supplement autotrophic carbon fixation with 38 heterotrophic capabilities similar to those in Kentron. We have shown that Kentron are the 39 only thiotrophic symbionts that appear to be entirely heterotrophic, unlike all other 40 thiotrophic symbionts studied to date, which possess either the Calvin-Benson-Bassham or 41 reverse tricarboxylic acid cycles for autotrophy. 42 Keywords: meiofauna, ectosymbiont, Gammaproteobacteria, protist, lithoheterotrophy 2 bioRxiv preprint doi: https://doi.org/10.1101/540435; this version posted February 5, 2019. The copyright holder for this preprint (which was not certified by peer review) is the author/funder, who has granted bioRxiv a license to display the preprint in perpetuity. It is made available under aCC-BY-NC-ND 4.0 International license. 43 Significance Statement 44 Many animals and protists depend on symbiotic sulfur-oxidizing bacteria as their main food 45 source. These bacteria use energy from oxidizing inorganic sulfur compounds to make 46 biomass autotrophically from CO2, serving as primary producers for their hosts. Here we 47 describe apparently non-autotrophic sulfur symbionts called Kentron, associated with marine 48 ciliates. They lack genes for known autotrophic pathways, and have a carbon stable isotope 49 fingerprint heavier than other symbionts from similar habitats. Instead they have the potential 50 to oxidize sulfur to fuel the uptake of organic compounds for heterotrophic growth, a 51 metabolic mode called chemolithoheterotrophy that is not found in other symbioses. 52 Although several symbionts have heterotrophic features to supplement primary production, in 53 Kentron they appear to supplant it entirely. 3 bioRxiv preprint doi: https://doi.org/10.1101/540435; this version posted February 5, 2019. The copyright holder for this preprint (which was not certified by peer review) is the author/funder, who has granted bioRxiv a license to display the preprint in perpetuity. It is made available under aCC-BY-NC-ND 4.0 International license. 54 Introduction 55 Chemosynthetic symbioses between heterotrophic, eukaryotic hosts and bacteria that use the 56 oxidation of inorganic chemicals or methane to fuel growth are common in marine 57 environments. They occur in habitats ranging from deep sea vents and seeps, where they are 58 responsible for much of the primary production, to the shallow water interstitial, where the 59 hosts are often small and inconspicuous meiofauna. Among the energy sources for 60 chemosynthesis are reduced sulfur species like sulfide and thiosulfate, and such sulfur- 61 oxidizing (thiotrophic) symbioses have convergently evolved multiple times (1). They are 62 commonly interpreted as nutritional symbioses where the symbionts fix CO2 autotrophically 63 into biomass with the energy from sulfur oxidation and eventually serve as food for their 64 hosts (1, 2). Indeed, several host groups have become so completely dependent on their 65 symbionts for nutrition that they have reduced digestive systems. All sulfur-oxidizing 66 symbioses investigated thus far possess a primary thiotrophic symbiont with genes of either 67 the Calvin-Benson-Bassham (CBB) (3–10) or reverse tricarboxylic acid (rTCA) (11, 12) 68 cycles for CO2 fixation, and the different pathways may relate to different ecological niches 69 occupied by the symbioses (13). The symbionts of the vestimentiferan tubeworms are 70 additionally able to encode both the CBB and rTCA cycles, which may be active under 71 different environmental conditions (14–16). Beyond sulfur oxidation and carbon fixation, 72 several thiotrophic symbionts have additional metabolic capabilities such as the uptake of 73 organic carbon (17), the use of carbon monoxide (18) and hydrogen (19) as energy sources, 74 and the ability to fix inorganic nitrogen (4, 5). 75 The thiotrophic ectosymbionts of the ciliate genus Kentrophoros constitute a distinct clade of 76 Gammaproteobacteria named “Candidatus Kentron” (hereafter Kentron) (20). Kentron has 77 previously been shown to oxidize sulfide and fix CO2 (21), and to be consumed and digested 4 bioRxiv preprint doi: https://doi.org/10.1101/540435; this version posted February 5, 2019. The copyright holder for this preprint (which was not certified by peer review) is the author/funder, who has granted bioRxiv a license to display the preprint in perpetuity. It is made available under aCC-BY-NC-ND 4.0 International license. 78 by its hosts (21, 22). Unlike most ciliates, which consume their food at a specific location on 79 the cell that bears feeding structures composed of specialized cilia, Kentrophoros has only 80 vestiges of such cilia, and instead directly engulfs its symbionts along the entire cell body 81 (23), suggesting that Kentron bacteria are its main food source. 82 Given that all previous studies of thiotrophic symbionts, including Kentron, have 83 characterized them as autotrophic, we expected that the pathways of energy and carbon 84 metabolism used by Kentron would resemble those in other thiotrophic bacteria involved in 85 nutritional symbioses. In this study, we used metagenomic and transcriptomic analyses of 86 single host individuals to show that the Kentron clade lacks the canonical pathways of 87 autotrophic CO2 fixation. Based on a metabolic reconstruction of the core genome from 88 eleven Kentron phylotypes collected from three different sites, and results from direct protein 89 stable isotope fingerprinting, we propose that it is a lithoheterotrophic nutritional symbiont, 90 relying on assimilation of organic substrates rather than fixation of inorganic carbon to feed 91 its hosts. 92 Results 93 Symbiont genome assemblies have high coverage and 94 completeness, and represent eleven phylotypes 95 Genomes of Kentron symbionts were binned from 34 metagenome assemblies, each 96 corresponding to a single Kentrophoros host ciliate individual. These samples represented 12 97 host morphospecies from three different geographical locations: the Mediterranean, 98 Caribbean, and Baltic Seas (Supplementary Table 1). The symbiont genome assemblies
Details
-
File Typepdf
-
Upload Time-
-
Content LanguagesEnglish
-
Upload UserAnonymous/Not logged-in
-
File Pages43 Page
-
File Size-