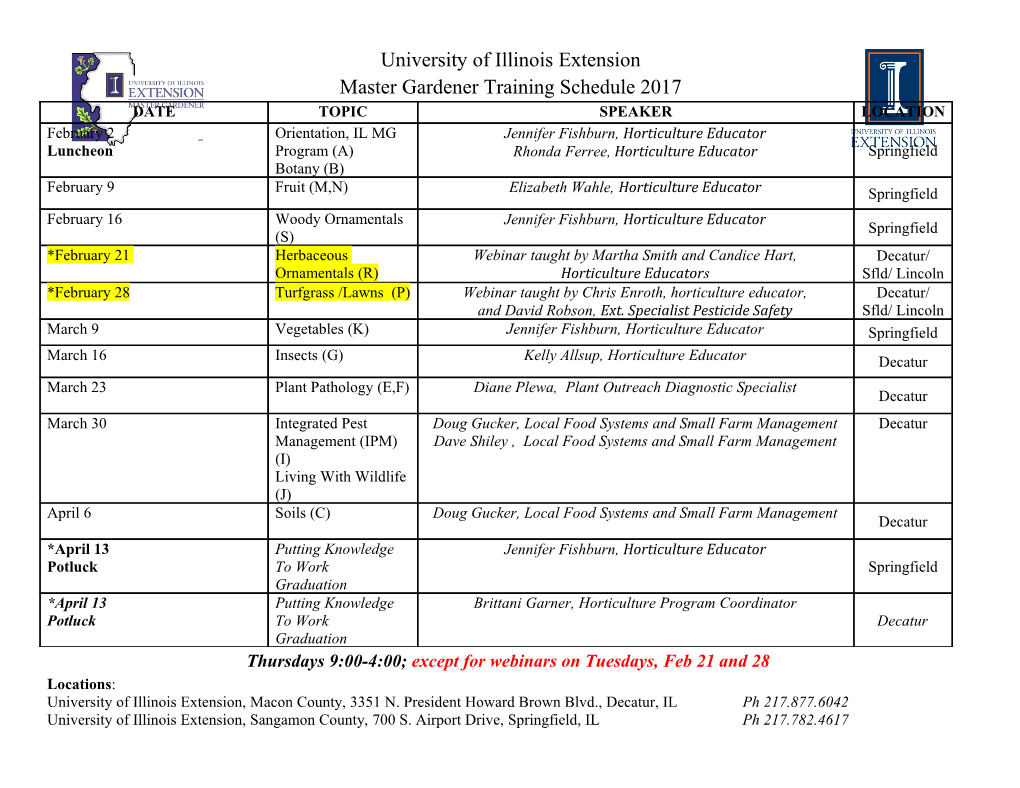
Master course: Star Formation and Origin of Planetary Systems Ewine F. van Dishoeck and Melissa McClure Leiden Observatory Spring 2020 Lecture 1: Introduction and overview www.strw.leidenuniv.nl/~vgelder/SPF/ 1.1 Motivation . Formation of stars and planetary systems is one of the most fundamental topics in astronomy but little material covered in bachelor curriculum . Many recent developments, both observationally and theoretically, including rapidly growing field of exoplanets . ‘Star formation spans densities from 104 cm-3 to 1024 cm-3, involves all the known forces of nature, with observational diagnostics across the entire spectrum, and requires experimental access to relevant primitive materials that has no parallel in any other branch of astrophysics’ Shu et al. 1993 1.2 Outline course . Discuss systematically the various stages in the formation of (low-mass) stars, from collapse of interstellar cloud to formation of planetary systems (see schedule) . Level: advanced course . Basic concepts will be briefly recapitulated as needed (e.g., interstellar clouds, line + continuum radiation, ….) . Expect self-study (e.g., generally no step-by-step derivations done in lectures) . Focus is on broad picture, not on doing problem sets . One aim: follow and put in context colloquia in this field . You have to tell us what you learned! From clouds to disks to planets and back Bill Saxton AUI/NRAO Organization . Lectures . Tuesday and Thursday Huygens 414 . Three afternoons for ‘werkcollege/problem sets’ . Tentamen . Oral exam on appointment . Short presentation to group as part of exam possible . If presentation on topic from first half of course, then exam on second part and vice versa . Required background . Radiation processes (Planck function, Einstein coeff.) . Statistical physics (Boltzmann, Maxwell distribution) . Quantum physics (H atom) . Assistants: Pooneh Nazari, Mantas Zilinskas, Martijn van Gelder Literature . Exam based on content of lecture notes . Background reading useful for better understanding . Review chapters listed at start of each chapter and linked to course website where available; references to specialized literature provided Useful reference books . Origin of stars and planetary systems (Crete II), ed. C.J. Lada & N.D. Kylafis (Kluwer) (1999) . Protostars & Planets series (Univ. Arizona) . PPVI: eds. H. Beuther et al. 2014 . PPV: eds. B. Reipurth et al. 2007 . Accretion processes in star formation, L. Hartmann (Cambridge) (2008) . Formation of stars, S. Stahler & F. Palla (Wiley) (2004) . Astrophysics of planet formation, P. Armitage (Cambridge) (2010) . See list on website for other books 1.3 Scenario for low-mass star formation Shu et al. 1987, 1993 The big picture: low-mass stars Shu et al. 1987 . Cores form within molecular clouds; gas and dust contract under their own gravitation and evolve toward centrally concentrated configuration such as a singular isothermal sphere (with 1/r2 density law) . Cloud core collapses from inside-out; protostar with surrounding disk forms at center; infalling envelope of gas and dust continues to rain down on growing star + disk, resulting in hundreds of magnitudes of extinction Figures: M. Hogerheijde Dense starless core B68 ESO-VLT Alves et al. 2001 Stars form in molecular clouds Carina nebula NASA/HST - Need long wavelengths to peer into dark clouds - Clouds form stars with efficiency of only a few%: why? The big picture (cont’d) . Stellar or disk wind breaks out along rotational axis of system, reversing infall and sweeping material into two outwardly expanding shells of gas and dust (‘jets, bipolar outflow’); infall still occurs in equatorial regions . Outflow angle opens up with time, terminating infall and revealing a newly formed star optically with a circumstellar disk (‘T Tauri phase’) Long wavelengths 3.5, 4.6, 8 µm Spitzer Space Telescope NASA/Spitzer Noriega-Crespo et al, 2004 - IR/Mm measures broadband thermal radiation from cold (10-50 K) and warm (50-300 K) dust particles and narrowband lines from molecules HH 30 disk + jet Optical image HST Jet Green: [O III] White: broadband Scattered stellar light Edge-on Disk Credit: C. Burrows/HST Characterizing stages of star formation with SED outflow n~104-105 cm-3 T~10 K infall Factor 1000 smaller Cloud collapse t=0 Protostar with disk t=105 yr Credit: M. McCaughrean Class I Spitzer 70 µm Spitzer 2MASS 6 7 Formation planets t=10 -10 yr Solar system t>108 yr Spectral energy distributon Characterizing stages star- and planet formation outflow 6 7 Formation planets t=10 -10 yr Solar system t>108 yr Cloud collapse t=0 Protostar with disk t=105 yr Disk Class II Star Class III 6 7 Formation planets t=10 -10 yr Solar system t>108 yr Note shiftSpitzer from probes long dust(far -atIR) temperatures to short (near between-IR) wavelengths 100 and 1500 with K. evolution Low-mass: Ophiuchus 3,.8, 24 µm Red: IR excess young stars Blue: mature stars The big picture (cont’d) . Planets form in circumstellar disks; disks are dispersed by winds, UV radiation, planet formation, and/or gravitational interactions with nearby stars . Mature and stable planetary system emerges Solar system view: Protosolar nebula Lunine 1990, and in PPIII First images of exoplanets Triple system Chauvin et al. 2005 Marois et al. 2008 ESO VLT Gemini Exoplanet statistics More than 4000 exoplanets discovered to date (radial velocity, Transit, Kepler!), some planetary systems (e.g., Trappist 1) Exoplanet.org, exoplanet.eu What sets diversity in exoplanets? Credit: Martin Vargic Super Earths, Mini Neptunes, Giant Planets, ... Low vs. high-mass star formation . Above scenario holds for formation low mass stars (<2 MSun), or ‘loosely aggregated’ systems ⇒ gravitational collapse occurs independently for individual small cores to form single stars or small multiples (binaries-quadruples) . High-mass star formation usually occurs in a ‘closely packed’ form, in which a large piece of a giant molecular cloud collapses to create a tight group of stars more or less simultaneously . Difference probably connected with initial mass of cloud, and strength of magnetic field and level of turbulence, which help to support cloud . Even for low-mass stars, alternative scenarios of turbulent core formation and fragmentation of filaments to form cores exist (see Lectures 2 and 3) . Galaxy-wide star formation relations (‘laws’) gaining increasing attention but not discussed here High-mass: Orion HST image Typical sizes Linear Size size Angular Taurus 140 pc Orion 450 pc 5 AU 0.04’’ 0.01” Inner disk 100 AU 0.7’’ 0.2” Outer disk 1000 AU 7” 2” Protostar envelope 10000 AU=0.05 pc 74” 23” Cloud core Note: debris disks found around nearby main sequence stars, ~10 pc => much bigger on the sky Typical spatial resolution mid/far IR/submm: 3-20”; only now ALMA → <0.1” 1.4 Some key historical developments (more details in individual chapters) . 17th cent: Many speculations about planets around other stars and possibility of life elsewhere . E.g. Huygens: Kosmotheoros 1698 . Dates back to Greeks, e.g. Ptolemy . ~1750: Swedenborg, Kant, Laplace postulate that solar system formed from ‘urnebel’ (‘solar nebula’) . 18th-20th cent: Observations of nebulae, dark clouds . E.g. W. Herschel: Orion 1789 . E. Barnard: catalogue of dark clouds 1919 . 1904-1930: Discovery of interstellar gas and dust . W. Hartmann: gas 1904 . Trumpler: dust 1930 Despite these observations/speculations, the prevailing view at the turn of the 20th century was that stars live forever and that origin of stars is part of cosmology History (cont’d) . 1950’s: Theory of stellar evolution ⇒ stars do not live forever, i.p. massive OB stars must have been formed recently . Schwarzschild, Hoyle, … . 1947: OB stars are members of stellar groupings (‘OB associations’). Space density too low to prevent disruption by Galactic tidal forces ⇒ must be young ⇒ star formation still ongoing . Ambartsumian 1947-1949; Blaauw 1946 History (cont’d) . 1960’s-1970’s: Mapping of interstellar clouds in H I (1960’s) and CO (1970’s)⇒ clouds contain much more mass than needed to form a single star or group of stars: abundant material available to form stars (e.g., Giant Molecular Clouds: up to 6 10 MSun) . 1960s-1970s: OB stars are near interstellar clouds with systematic (?) age variations ⇒ sequential/triggered star formation . Blaauw 1964, Elmegreen & Lada 1976 Sequential/triggered star formation C. Lada 1999 Crete II History (cont’d) . 1950’s-1960’s: Discovery of late-type stars with H emission lines ⇒ low-mass pre- main sequence stars: T Tauri, Herbig Ae/Be stars . e.g., Joy 1945, Walker 1956, Herbig 1960 . 1960’s-1970’s: Theories of pre-main sequence evolution and cloud collapse . e.g., Hayashi 1961, Larson 1969, Shu 1977 History (cont’d) . 1970’s-1980’s: Discovery of Herbig-Haro objects with high proper motions (optical jets) and bipolar outflows (CO) . E.g., Luyten 1971, Cudworth & Herbig 1979, Snell et al. 1980, Lada 1985 . 1980’s: IRAS satellite maps clouds at 12, 25, 60, 100 µm ⇒ distribution of young stellar objects (YSOs) in clouds and evolutionary sequence . E.g., Beichman et al. 1983, Lada & Wilking 1984, Adams, Shu & Lada 1987 History (cont’d) . Early 1980’s: Discovery of tenuous ‘debris’ disks around mature main-sequence A stars . Vega: Aumann et al. 1984, IRAS . β Pic: Smith & Terrile 1984: optical + coronagraph . Late 1980’s, early 90’s: Development of ground- based infrared and submm observations ⇒ indirect evidence for massive disks around young T Tauri stars . Mid 1990’s: Definite proof for existence of disks around young stars through mm interferometry and HST imaging (‘proplyds’) Beta Pictoris disk Disk warp ⇒ Planet? Planet confirmed by direct imaging Credit: ESO Lagrange et al. 2010 Protoplanetary disks Size disks ~1010 km = 2xSun-Pluto Give me matter and I will make a world out of it Kant (1755) HST/C. O’Dell History (cont’d) . 1970’s-1990’s: Development of theories for dust coagulation, planet formation, early solar nebula .
Details
-
File Typepdf
-
Upload Time-
-
Content LanguagesEnglish
-
Upload UserAnonymous/Not logged-in
-
File Pages56 Page
-
File Size-