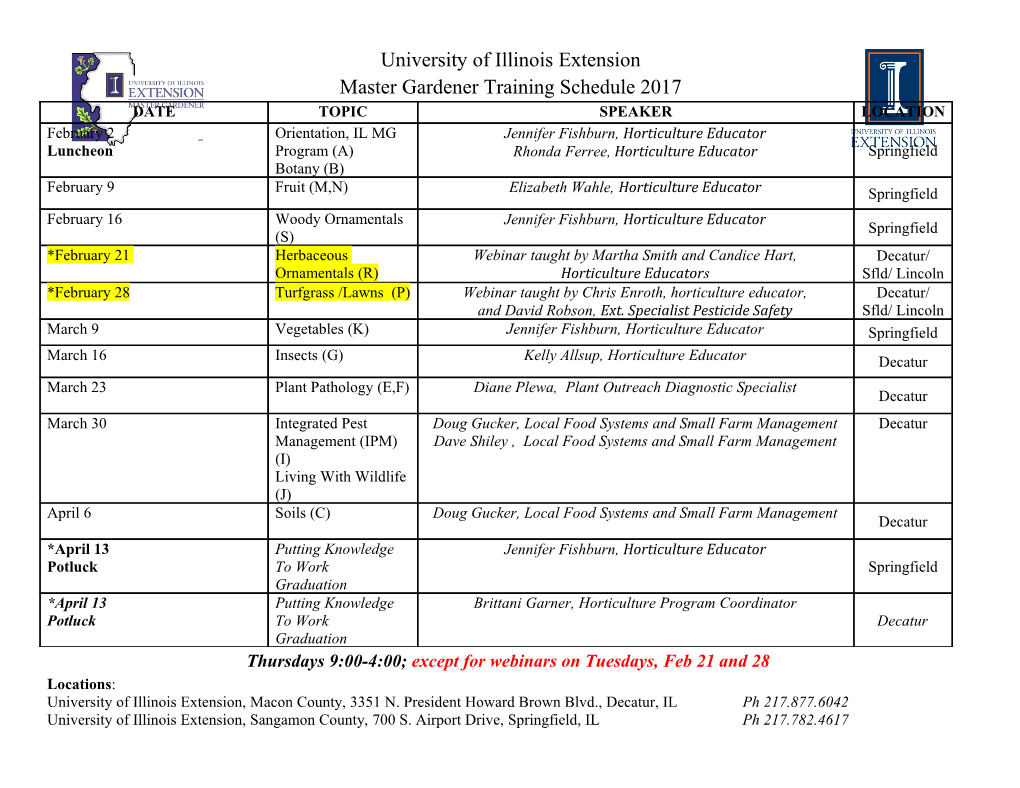
https://doi.org/10.1130/G48523.1 Manuscript received 21 July 2020 Revised manuscript received 21 October 2020 Manuscript accepted 11 January 2021 © 2021 Geological Society of America. For permission to copy, contact [email protected]. Small-world dynamics drove Phanerozoic divergence of burrowing behaviors Andrea Baucon1,2*, Carlos Neto de Carvalho2,3, Fabrizio Felletti4, Gabriele Tosadori5 and Alexandre Antonelli6,7, 8 1 Dipartimento di Scienze della Terra dell’Ambiente e della Vita (DISTAV), Genova University, Corso Europa 52, 16132 Genoa, Italy 2 Geology Office of C.M. Idanha-a-Nova, Naturtejo UNESCO Global Geopark, Avenida Zona Nova de Expansão, 6060-101 Idanha- a-Nova, Portugal 3 Instituto Dom Luiz, Department of Geology, Lisbon University, Campo Grande, 1749-016 Lisbon, Portugal 4 Department of Earth Sciences, Milan University, Via Mangiagalli 34, 20133 Milan, Italy 5 Department of Medicine, Verona University, Policlinico GB Rossi, Piazzale L.A. Scuro 10, 37134 Verona, Italy 6 Royal Botanic Gardens, Kew, Richmond TW9 3AE, UK 7 Gothenburg Global Biodiversity Centre, Department of Biological and Environmental Sciences, University of Gothenburg, Carl Skottsbergs gata 22 B, 413 19 Göteborg, Sweden 8 Department of Plant Sciences, University of Oxford, South Parks Road, Oxford OX1 3RB, UK ABSTRACT (Seilacher, 1953), hydrocarbon exploration (La Species of burrowing animals have changed substantially over evolutionary time scales, Croix et al., 2013, 2012; Bednarz and McIlroy, but, surprisingly, burrows display persisting morphological patterns throughout the Pha- 2015), scientific drilling, and characterization nerozoic. Deep-sea burrows are geometrically patterned, whereas shallow-marine burrows of aquifers (Droser and O’Connell, 1992; Cun- display simpler morphologies. This divergence between burrow associations is one of the ningham et al., 2009). Ethology is central to central conundrums of paleontology, but it has never been quantitatively demonstrated, and this framework because trace fossils are mani- the organizing principles responsible for this structure remain unknown. We show that the di- festations of behavior, which is controlled by vergence of burrow associations has been shaped by small-world dynamics, which is proposed the environment; environmental parameters, as a major macroevolutionary force in marine environments. Using network analysis, our in turn, are strongly affected by water depth study reveals that the association patterns between burrow morphotypes in 45 paleontological (Seilacher, 1953; Ekdale, 1988; MacEachern sites span ∼500 m.y. Strong statistical support is demonstrated for a surprising association et al., 2012). pattern, according to which the data set is optimally partitioned into two subgroups of tightly Despite its popularity and intuitive recog- associated burrow types. These groups correspond to shallow- and deep-marine biomes. Our nition, the divergence hypothesis has never analysis demonstrates that across the Phanerozoic Eon, burrows did not assemble randomly been quantitatively tested, and the organizing nor regularly, following instead small-world assembly rules remarkably similar to those that principles that underpin it remain unknown. shape human social networks. As such, small-world dynamics deeply influenced gene flow This issue is addressed here by analysis of as- and natural variation in heritable behavior across evolutionary time. sociation patterns of Phanerozoic trace fossils. Our study tests the specific predictions of the INTRODUCTION hypothesis states that deep-sea associations of divergence hypothesis that (1) association pat- Species of burrowing organisms have trace fossils have retained their distinctiveness terns of trace fossils are inhomogeneous, i.e., changed dramatically throughout the Phanero- from shallow-marine trace fossils throughout trace fossils are associated neither randomly nor zoic, which comprises the past 541 m.y. of bio- most of the Phanerozoic (Seilacher, 1953, 1967, regularly; and (2) any trace-fossil association is logical evolution on Earth. Nevertheless, bur- 1978, 2007). In other words, the hypothesis as- related to a specific biome, i.e., shallow or deep rows and other traces of organism-substrate serts that deep-sea trace fossils are morphologi- marine. Accordingly, the first null hypothesis interactions (e.g., trails, trackways) have main- cally different from shallow-marine trace fossils is that trace fossils are randomly associated; tained similar morphologies throughout the (Fig. 1). the second is that they are regularly associated; same time span, with each morphology being The quantum leap in the understanding the third is that there is no correlation between associated with a specific environmental setting of life-substrate interactions imparted by this groups of associated traces and their environ- (Frey and Seilacher, 1980). In fact, one of the observation (MacEachern et al., 2012) led to mental setting. fundamental hypotheses of paleontology con- the recognition of associations of trace fos- cerns the Phanerozoic divergence between shal- sils across time and space (i.e., ichnofacies; METHODS low- and deep-sea trace fossil associations. This Seilacher, 1953, 1967). As a consequence of The ideal source data for quantitatively testing this recognition, trace fossils became a central the divergence hypothesis are the data from which *E-mail: [email protected] tool in the reconstruction of ancient ecosystems the divergence idea first stemmed (Seilacher, CITATION: Baucon, A., et al., 2021, Small-world dynamics drove Phanerozoic divergence of burrowing behaviors: Geology, v. 49, p. XXX–XXX, https://doi. org/10.1130/G48523.1 Geological Society of America | GEOLOGY | Volume XX | Number XX | www.gsapubs.org 1 Downloaded from http://pubs.geoscienceworld.org/gsa/geology/article-pdf/doi/10.1130/G48523.1/5259222/g48523.pdf by GSA Content on 26 March 2021 A D Figure 1. Phanerozoic BC divergence hypothesis. EF It predicts that simple burrows (A–C) are found in shallow-sea deposits, and geometrically pat- terned burrows (D–F) in deep-sea settings. Scale bars are 1 cm wide. (A) Cruziana (Ordovician). (B) Asteriacites (Triassic). (C) Diplocraterion (Jurassic). (D) Nereites (Cretaceous). (E) Paleodictyon (Creta- ceous). (F) Spirophycus (Cretaceous). See Table S1 (see footnote 1) for specimen details. 2007; Table S2 in the Supplemental Material1). that are equivalent; i.e., with the same number ichnofossil network topology. The seafloor was This data set includes presence-absence data of nodes and average degree. We empirically simulated by a unidimensional domain across of environmental proxies and 32 taxa of trace generated 1000 random networks using the which ichnotaxa are distributed. The spatial fossils (ichnotaxa) in 45 sites, ranging from Erdős and Rényi (1959) model (Fig. S1A in range of each taxon can change at discrete points Cambrian to Miocene in age. Each assemblage the Supplemental Material). To test the third in time according to a defined set of rules: (1) a of the data set represents the work of animals that hypothesis, subgroups are searched by using only “specialist rule”, i.e., keeping a narrow spatial lived in the same biome, but it is not necessarily the information encoded in the topology of the range across time; or (2) a “generalist rule”, i.e., an ichnocoenose. Using the framework of ichnofossil network; then the correlation between keeping a wide range for the entire duration of network analysis (Baucon and Felletti, 2013; the detected subgroups and environmental the simulation. At the end of the simulation, a Baucon et al., 2014, 2015), the source data table proxies is tested. To detect subgroups, we applied network was produced based on co-occurrence is translated into a network by representing the concept of modularity, which measures the relationships between taxa, and the network was ichnotaxa as nodes and by connecting those taxa number of links falling within subgroups minus compared to the ichnofossil network. Detailed that co-occur in at least one paleontological site. the expected number in a random null model methods and definitions are provided in the The resulting network (Fig. 2), herein named (Newman, 2006; Fortunato, 2010). By optimizing Supplemental Material. the ichnofossil network, is made of 32 nodes modularity over possible subgroups of the studied (ichnotaxa), each of which is linked to 19.8 taxa network, we determined whether there exist any RESULTS AND DISCUSSION on average (average degree). To test the first natural partitions of its nodes (Newman, 2006; Ichnofossil Network versus Random and and second null hypotheses, we compared the Blondel et al., 2008). We then evaluated whether Regular Null Models topological properties of the ichnofossil network ichnotaxa of each subgroup have a higher In the ichnofossil network, any two nodes with those of random and regular null models probability of being associated with shallow- are connected by a minimum number of links marine proxies versus deep-marine proxies. The (distance). For instance, the distance between probability of association was quantified using Cosmorhaphe and Spirophycus is 1 because 1Supplemental Material. Supplemental text, the Jaccard similarity and a χ2 test (Hammer and the corresponding ichnotaxa are found in the 7 supplemental figures, 3 supplemental tables, Harper, 2006). same paleontological site(s) (Fig. 2). This im- and 4 files of Python code. Please visithttps:// doi.org/10.1130/GEOL.S.14120408 to access We propose
Details
-
File Typepdf
-
Upload Time-
-
Content LanguagesEnglish
-
Upload UserAnonymous/Not logged-in
-
File Pages5 Page
-
File Size-