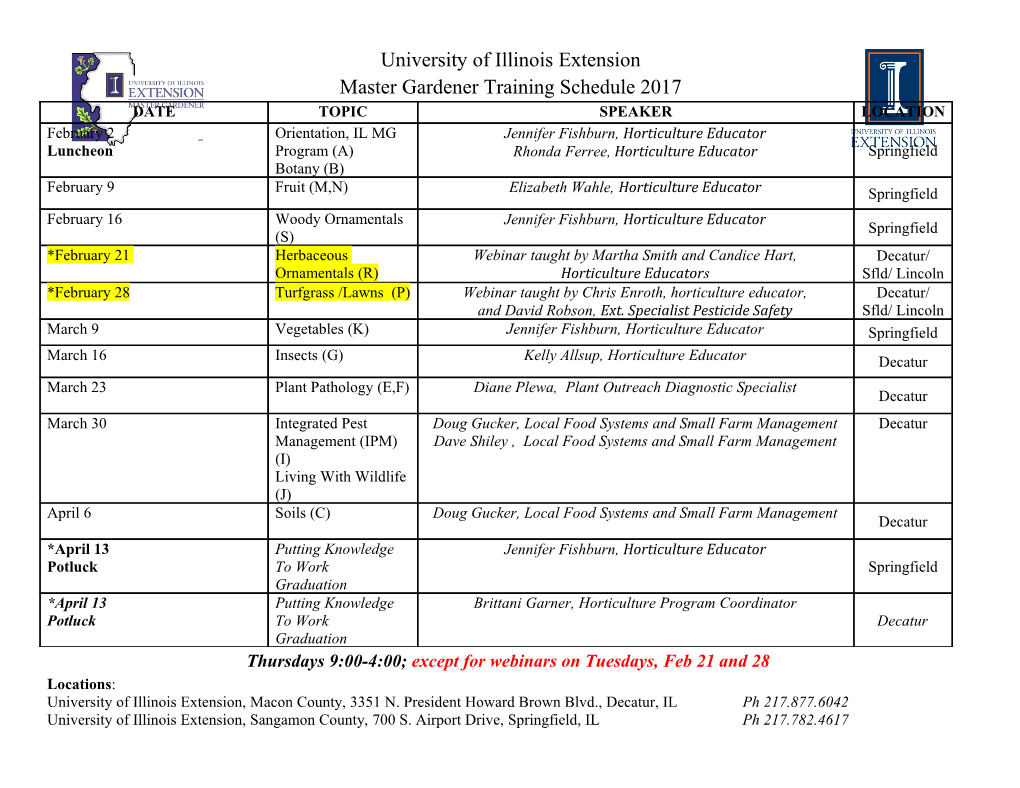
Spectroelectrochemical determination of the redox potential of pheophytin a, the primary electron acceptor in photosystem II Yuki Katoa, Miwa Sugiurab, Akinori Odaa, and Tadashi Watanabea,1 aInstitute of Industrial Science, University of Tokyo, 4-6-1 Komaba, Meguro-ku, Tokyo 153-8505, Japan; and bCell-Free Science and Technology Research Center, Ehime University, Bunkyo-cho, Matsuyama, Ehime 790-8577, Japan Edited by James Barber, Imperial College London, London, United Kingdom, and accepted by the Editorial Board August 20, 2009 (received for review May 15, 2009) Thin-layer cell spectroelectrochemistry, featuring rigorous poten- estimated from the potentials of PS II electron acceptors (10–12) tial control and rapid redox equilibration within the cell, was used or assessed by computation (13–17). ؊ to measure the redox potential Em(Phe a/Phe a ) of pheophytin In the former case, the Phe a redox potential Em(Phe a/Phe (Phe) a, the primary electron acceptor in an oxygen-evolving aϪ), together with the P680* Ϫ Phe a free energy difference, is ϩ photosystem (PS) II core complex from a thermophilic cyanobac- a good clue to estimate Em(P680/P680 ). An attempt in this terium Thermosynechococcus elongatus. Interferences from dis- direction was made in 1979 by Klimov et al. (11), who had solved O2 and water reductions were minimized by airtight sealing detected Phe a as the primary electron acceptor in PS II of the sample cell added with dithionite and mercury plating on the membranes from pea (18). They obtained Ϫ610 Ϯ 30 mV as Ϫ gold minigrid working electrode surface, respectively. The result Em(Phe a/Phe a ) and, using the P680* Ϫ Phe a energy ؊ -difference of Ϸ80 meV from delayed fluorescence measure ؍ ( obtained at a physiological pH of 6.5 was Em(Phe a/Phe a ϩ ؊505 ؎ 6 mV vs. SHE, which is by Ϸ100 mV more positive than the ments (19), estimated the Em(P680/P680 ) value to be ϩ 1,120 Ϯ values measured Ϸ30 years ago at nonphysiological pH and widely 50 mV (11). In 1981 Rutherford et al. (20) indirectly assessed the Ϫ accepted thereafter in the field of photosynthesis research. Using Em(Phe a/Phe a ) value from an EPR study of the P680 triplet BIOPHYSICS AND ؊ Ϫ the P680* Phe a free energy difference, as estimated from kinetic states, to obtain 604 mV, being close to that by Klimov et al. COMPUTATIONAL BIOLOGY analyses by previous authors, the present result would locate the For almost three decades thereafter, the values of approximately ؉ Ϫ Ϫ ϩ Em(P680/P680 ) value, which is one of the key parameters but still 600 mV for the Em(Phe a/Phe a ) and approximately 1.1 V ϩ -resists direct measurements, at approximately ؉1,210 mV. In view for Em(P680/P680 ) have been widely accepted in the discus of these pieces of information, a renewed diagram is proposed for sions on PS II energetics, with no further attempts to measure Ϫ the energetics in PS II. Em(Phe a/Phe a ). Recently Rappaport et al. (12) disputed the validity of the Ϫ charge separation ͉ photosynthesis ͉ spectroelectrochemistry ͉ Em(Phe a/Phe a ) value, on the ground that the Phe a Ϫ QA water oxidation potential difference did not fit their kinetic analytical data. A value of Ϫ80 mV or Ϫ30 mV in the presence of DCMU (an n the photosynthetic primary process of higher plants, algae, inhibitor blocking electron transfer from QA to the second plastoquinone QB) has been assumed to be reasonable for Iand cyanobacteria, photosystem (PS) I and PS II cooperate in Ϫ series to convert photon energy into chemical energy through Em(QA/QA ) since the thorough studies of Krieger et al. (21–23), giving the Phe a Ϫ QA potential difference of Ϸ600 meV, light-induced charge separation and subsequent electron trans- Ϫ although scattered Em(QA/QA ) values in three ranges, approx- fers. PS II appears to catalyze water oxidation at a pentanuclear Ϫ Mn Ca cluster that accumulates oxidizing equivalents (see re- imately 250, 0, and 125 mV, were reported (reviewed in ref. 4 21). However, the fluorescence decay data by Rappaport et al. cent reviews in refs. 1–5). It is generally supposed that the water Ϫ oxidation is triggered by charge separation between the primary on the charge recombination of QA at the S2 state in the presence of DCMU in Synechocystis PCC 6803 mutants PS II electron donor, P680, and the primary electron acceptor, pheo- ϩ Ϫ indicated that the Phe a Ϫ QA potential difference should be phytin (Phe) a. The initial radical pair P680 Phe a formed by ϩ smaller by Ϸ250 mV. They then estimated the Em(P680/P680 ) the charge separation, drives forward electron transfer from Phe Ϫ Ϫ ϩ value to be ϩ1.26 V (12) on the basis of E (Q /Q ) and kinetic a to the first plastoquinone Q , and hole transfer from P680 m A A A analytical data in the presence of DCMU. Later, Grabolle and to the Mn Ca cluster through a redox-active tyrosine residue 4 Dau (10) analyzed the delayed and prompt Chl fluorescence of denoted Y , thus preventing charge recombination. Recent Z PS II membranes in the absence of inhibitor and assessed the X-ray crystallography clarified the arrangement of protein sub- E (P680/P680ϩ) value to be ϩ1.25 V, by citing E (Q /Q Ϫ)to units and cofactors in PS II with 2.9–3.7-Å resolution (6–9), m m A A be Ϫ80 mV. Since then, the value of approximately ϩ1.25 V is visualizing the electron transfer pathway. ϩ generally accepted for E (P680/P680 ) (1–5) and is used as a To date, the nature of P680 remains controversial. Available m reference in computational chemistry (13–16). experimental data and theoretical analyses suggest that P680 is In view of these finding, a renewed attempt to determine the assignable to the pigment cluster of the four chlorophyll a Ϫ Em(Phe a/Phe a ) value would be of much significance. Actually, molecules (denoted PD1,PD2, ChlD1, and ChlD2) in the PS II reaction center (1, 5). Further uncertainty surrounds the value ϩ of the redox potential Em(P680/P680 ). Although the Em(P680/ Author contributions: Y.K. and T.W. designed research; Y.K., M.S., and A.O. performed ϩ P680 ) value is an essential parameter to draw a whole picture research; Y.K. analyzed data; and Y.K., M.S., and T.W. wrote the paper. of the PS II energetics, its direct measurement has never been The authors declare no conflict of interest. attained (10) because water, present as dominant (solvent) This article is a PNAS Direct Submission. J.B. is a guest editor invited by the Editorial Board. molecules in most sample solutions, is oxidized first heavily and 1To whom correspondence should be addressed. E-mail: [email protected]. tends to mask the subsequent oxidation of the target entity P680 This article contains supporting information online at www.pnas.org/cgi/content/full/ ϩ at a higher potential. The Em(P680/P680 ) value has hence been 0905388106/DCSupplemental. www.pnas.org͞cgi͞doi͞10.1073͞pnas.0905388106 PNAS Early Edition ͉ 1of6 Downloaded by guest on September 25, 2021 a number of questions have been raised (ref. 12; see also 0.010 Ϫ 450 Discussion below) as to the reliability of the Em(Phe a/Phe a ) measurements reported only in the 1978–1981 period (11, 20), 0.005 carried out with a chemical titration mode at nonphysiological pH values to render the H2O/H2 equilibrium potential negative . u enough (24, 25): Klimov et al. performed the measurement while . 0 a changing the pH of a solution from 8 to 11 (11) and Rutherford / 517 547 et al. adjusted the pH at 11 through the titration (20). A –0.005 Spectroelectrochemistry is a powerful means to probe into the ∆ redox behavior of biological molecules (26) because it (i) re- –0.010 quires only a small amount of sample, (ii) allows for strict potential control, and (iii) facilitates rapid redox equilibration within a sample cell. These features enabled us to determine the –0.015 428 redox potential Em of P700 (primary donor in PS I) and other 400 450 500 550 600 components to within Ϯ2 mV (27–31) and to unveil the species Wavelength / nm dependence of the Em value of P700 (28, 29). This has been Ϫ extended in the present work to measure the Em(Phe a/Phe a ) Fig. 1. Light-minus-dark difference spectra demonstrating the photoaccu- value in PS II complexes at physiological pH, by overcoming mulation of Phe aϪ in the PS II complexes in the OTTLE cell, where the potential several difficulties inherent in the strongly negative potential of the working electrode was set at Ϫ350 mV (solid curve) and Ϫ500 mV range. Use of electrode material exhibiting a large hydrogen (dashed curve). overpotential, of which mercury is a typical example, has a possibility to poise solution potential at values more negative than possible by titration with chemical reductants (24, 25). The after a measurement at Ϫ500 mV, the light-induced difference Ϫ Em(Phe a/Phe a ) value thus obtained is compared with the spectrum was almost identical with the counterpart shown in Fig. previous value and kinetic analytical data, and the energetics for 1A; this ensures that the redox reaction of Phe aD1 is fully Ϫ PS II is discussed in the light of the renewed Em(Phe a/Phe a ) reversible. value. Fig. 2 gives the time courses of absorbance change at 450 nm during and after continuous illumination (photoaccumulation of Results Phe aϪ) at a series of negative potentials applied to the working In this work, we used PS II core complexes from a thermophilic electrode.
Details
-
File Typepdf
-
Upload Time-
-
Content LanguagesEnglish
-
Upload UserAnonymous/Not logged-in
-
File Pages6 Page
-
File Size-