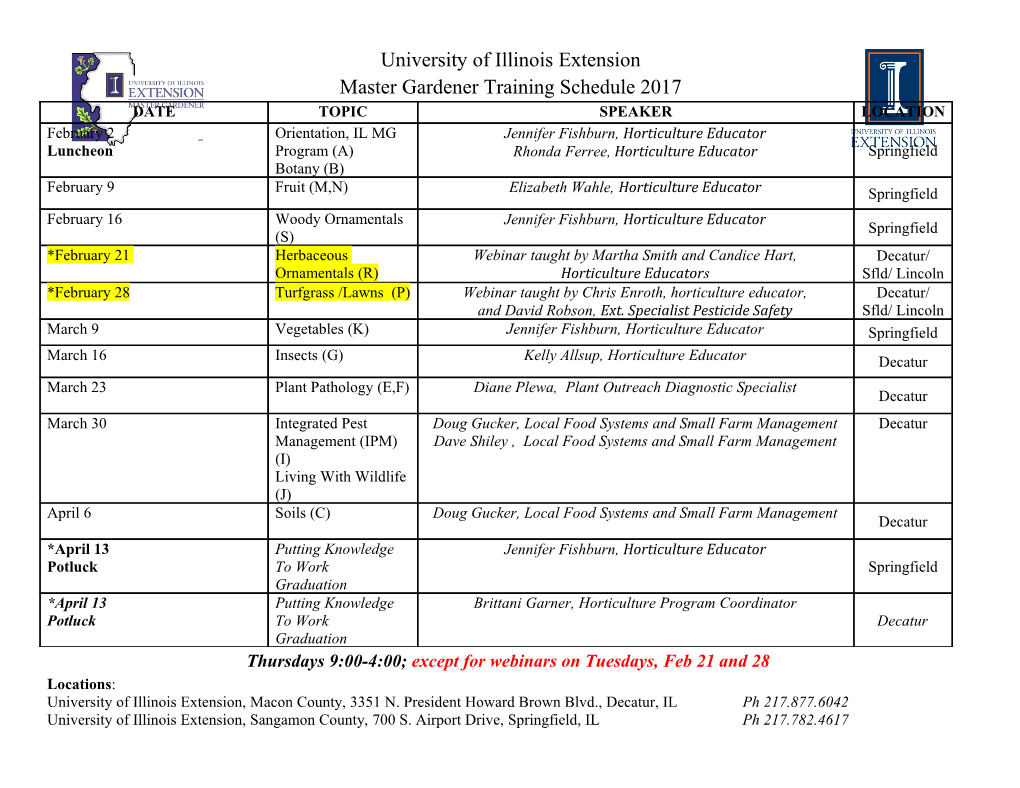
Materials Design Principles of Ancient Fish Benjamin Bruet, Juha Song, Mary C. Boyce Christine Ortiz,* Associate Professor *Department of Materials Science and Engineering, MIT Materials of Interest Exoskeletal Tissues Musculoskeletal Tissues Seashell nacre (Bruet, et al. JMR 2005) Cartilage (Han, et al. Biophys. J. 2007) Berkovich diamond probe tip 1 mm 71º PCM Cell c 8 μm 500 nm 0.8 μm Armored Fish Bone (Tai, et al. Nature Materials 2007) 50 nm Motivation Musculoskeletal Tissues (Medical) • The degradation of load-bearing musculoskeletal tissues during aging, injury, and / or disease affects millions of people worldwide. • The application of experimental and theoretical nanotechnologies to the field of musculoskeletal tissues and tissue engineering holds great promise for significant and rapid advancements towards tissue repair and/or replacement and even possibly a cure for people afflicted with ailments such as osteoarthritis. Exoskeletal Tissues (Engineering) (Ashby, 1999) • Inspiration and guidance for improved and increasingly advanced structural engineering materials; e.g. body armor and vehicle protection for defense and security applications. • Learning the basic principles of “mechanical property amplification" via complex, hierarchical and heterogeneous nanostructures that undergo a wide variety of deformation mechanisms at multiple length scales. Comparison of natural and historic engineered armor Tomasz Arciszewski and Joanna Cornell I.F.C. Smith (Ed.): EG-ICE 2006, LNAI 4200 Natural armor design : Maximize survivability Maximum Protection Maximum Mobility Tradeoff 1. Maximize energy absorption 1. Maximize flexibility 2. Maximize energy dissipation 2. Decrease Weight 3. Minimize deformations 4. Minimize penetration 5. Maximize visual impact 6. Maximize noise/sound impact -Metabolic costs; - materials available in the environment -Hygiene : lack of flexibility may increase vulnerability to parasites -Thermoregulation Tomasz Arciszewski and Joanna Cornell I.F.C. Smith (Ed.): EG-ICE 2006, LNAI 4200 Today’s human body armor Maximum Maximum Mobility Protection/Survivability Tradeoff Modern armors exist which can protect a person against even the high-velocity rounds fired by assault, battle or sniper rifles→too bulky and heavy for practical use 1. Flat plate inserts to protect vital organs only -The heart, lungs, central nervous systems, etc. are usually well protected, Helmet to Not Mobile Enough & Not Effective Against Shrapnel protect head. Limbs rarely armored or Blast 2. Weight reduction desirable. 3. Minimal protection from blast (improvised explosive devices, IED’s) 4. Multi-hit capability desirable. Today’s human body armor ; Fort Polk, Louisiana Gareth McKinley Christine Ortiz Greg Rutledge Karen Gleason John Joannopolous Ned Thomas Today’s human body armor – Fort Polk, Louisiana Polypterus senegalus : An ancient armored fish 23 Turning maneuver 1 (total time: 2.6 s)3 • Dates back to the Cretaceous period > 60 million yrs ago • Lives in freshwater, swampy, shallow floodplains and estuaries in Africa • Has a rudimentary lung (“swim bladder”) which allows them to leave the water for extended periods (1-2 days) • Territorial fighting; prone to in-fighting with its own species and conspecifics; jaw structure and skull are capable of supporting powerful bites1 • Interlocking, quad-layered mineralized armored scales while still maintaining body flexibility and swimming at high speeds2,3 1Kodera et al. (1994), 2Gembella et al. (2002), 3Tytell et al. (2005) Microscale geometry of P. senegalus armored scales and joints SEM image of scale top outer surface SEM image of peg (p) and socket (so) joint Gemballa, J. Morph. 2002 Cross-sectional multilayered structure of P. senegalus mineralized fish scales 3 1 2 1 mm Ganoine 100 μm Dentin • Quadlayered microstructure where each material is an organic-inorganic bionanocomposite1 Isopedine -Ganoine : outer layer, highly mineralized Bone 10 μm (>95%), noncollagenous enamel2,3 -Dentin : collagen / nanocrystalline apatite, reduced mineral content compared to ganoine5,6 -Isopedine : microstratified collagenous layers4 -Bone : collagenous lamellae5 1Sire (1990), 2Ørvig (1967), 3, 4Meunier (1980, 1987), 5Daget et al. (2001), 6Nalla et al. (2005) Mineral content of cross-section of P. senegalus fish scales Back scattered electron (BSEM) microscopy image Curved ganoine geometry, differentiated dentin thickness : capable of better isopedine energy absorption, deformations of a shell structure are bone smaller than those of a flat surface under the same impact force. Ultrastructure of ganoine from P. senegalus scales ● Tapping mode AFM of the ganoine surface showed dense array of hemispherical nanoasperities (diam ~ 50-100nm, height ~20nm) ● Higher magnification SEM imaging of the ganoine showed nanocrystals, hydroxyapatite (diameter ~50 nm, length 100’s of nm), consistent with the nanoasperity size found at surface. SEM image of cryo-fractured ganoine Ultrastructure of ganoine from P. senegalus scales outer surface 1 tubercule SEM image of a cryo-fractured cross-section of the ganoine Back-scattered electron microscopy of ganoine- dentin interface in P. senegalus scales ganoine ● Unique corregated microstructure, spatially Heterogeneous stresses and a higher net interfacial compression which could serve to prevent delamination dentin Sub-microlayered structure of isopedine in P. senegalus scales high mineral content Crack arresting at ganoine-dentin interface in P. senegalus scales Back scattered electron (BSEM) microscopy image Objectives : Materials design principles of P. senegalus mineralized fish scales •To quantify the local mechanical properties (e.g. modulus, yield stress) spatially through the four material layers in the cross-section of an individual scale and to establish structure-property relationships of each layer •To incorporate these results into a larger-scale computational model of the entire scale to determine the effect of local spatial variations, e.g. gradients, etc. on larger scale biomechanical properties; in this case penetration resistance Ganoine 3 Ganoine Dentin 2 1 Dentin Isopedine Isopedine Bone Bone 10 μm x x xxxxxxx x x •To relate multiscale structure-property design relationships to biomechanical function Methods : Mechanical properties of the four material layers in individual P. senegalus scales • Estimation of indentation modulus & hardness1 using instrumented nanoindentation Berkovich 500 3 400 E Maximum Load O-P 2 N) S N) H 1 μ (500 μN) μ 300 O-P Dentin Load ( Load 200 Indenter Load ( 100 200 nm 3 μm 0 (RTip ~ 275 nm) 0 20 40 60 80 100 120 140 Depth (nm) fish scale Depth (nm) • Determination of yield stress via finite element analysis (FEA) - Elastic perfectly-plastic using 4-node bilinear axisymmetric quadrilateral elements Indenter Tip - Tip area function (TAF) of 2D tip is as same Indented Material as one of 3D experimental Berkovich tip 3 2 1Oliver & Pharr (1992) 1 50 μm Multilayered and graded mechanical properties of four material layers of an individual P. senegalus scale inner Isop. surface Gan. Dent. 1 3 N) 2 μ 80 5 Bone Modulus Bone Isopedine Ganoine O-P Modulus Bone IsopedineGanoine 4 60 Hardness Load ( O-P Hardness Dentin Dentin 3 40 Epoxy 2 ` 20 1 ModulusModulus (GPa) (GPa) Hardness (GPa) (GPa)Hardness Hardness Depth (nm) 0 0 O-P Modulus (GPa) O-P Hardness (GPa) 0 50 100 150 200 250 300 350 400 • Layers ordered from the outer stiffest and DistanceDistance (μ m)(μm) hardest surface (ganoine) to the most Junction compliant and softest inner (bone) Ganoine • Negative Gradation within ganoine and Isopedine Dentin dentin layers; no measurable gradation in isopedine and bone •FEA shows good agreement with exp. data 330 340 350 360 370 380 390 400 410 420 330 340 350 360 370 380 390 400 410 Distance (μm) (σY ~ 2000, 400, 215 and 180 MPa from outer to inner layers) AFM imaging of residual indents in each layer Dent. Isop. Phase Image Ganoine Isop. Bone • Each layer exhibits residual plastic deformation at 500 μN • Nanoasperities appear flattened in the indented area ) Y x σ xxx Isop Dent Bone Junction xxxxxxxxxx Junction xx Gan Gradient DistanceDistance ((μμm)m) 25 0 25 50 50 7575 100 100 380380 400 20002000 m 1500 m μ μ 5 5 elastic-perfectly plastic uivalent of Vickers tip uivalent of 1000 m μ m μ 3 500 3 400 Yield Strength(MPa) 215 180 tation) material properties (E, 1800 Yield strength (MPa) m m m m μ μ μ μ 8 46 46 45 300 60 e compared to All Ganoine, 55 50 m m μ μ 40 5 5 All Dent All Bone Discrete Gradient 30 All Gan 2525 m μ m Modulus (GPa) Modulus 20 μ 3 14.5 3 13.5 10 O-P Modulus (GPa) 00 2525 50 75100 100 380380380400 400 DistanceDistance ((μμm)m) provides an axisymmetric eq 2 3 1 xxxxx m Gan Isop irtual microindentation multilayered scale of cross-section using finite element analysis Bone Dent μ Discrete V 20 2D conical tip All Dentin and Bone models. incorporated xxxxxx (used experimentally). • and gradient models ar Discrete • material of each layer is assumed to be The • determined (nanoinden Experimentally • Virtual microindentation of multilayered scale cross-section using finite element analysis Microlayering provides the effective mechanical penetration resistance of the entire scale Gradient Discrete P 0.5 All Dentin surface 0.4 All Ganoine 0.3 3 Fish scale All Bone 1 2 0.2 P, Load (N) 0.1 0.0 0 123456 7 δ, Depth (μm) •Multilayer P-δ exhibits ganoine behavior up to P = ~ 0.1 N (~1 μm) • Multilayer, at P > 0.1 N, is more compliant than ganoine •Multilayer P –δ substantially stiffer and harder than dentin • Discrete and
Details
-
File Typepdf
-
Upload Time-
-
Content LanguagesEnglish
-
Upload UserAnonymous/Not logged-in
-
File Pages48 Page
-
File Size-