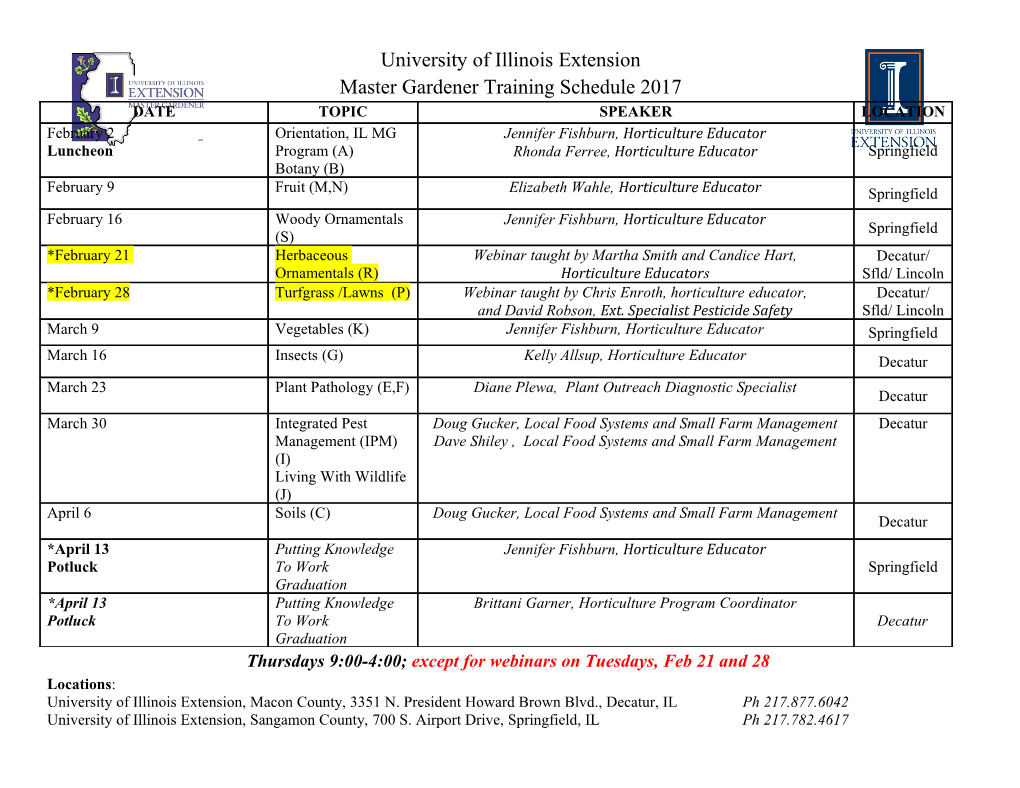
Health Science Campus FINAL APPROVAL OF DISSERTATION Doctor of Philosophy in Biomedical Sciences Translational Control in Escherichia coli: Hfq and PvuII Submitted by: Meenakshi Kaul Kaw In partial fulfillment of the requirements for the degree of Doctor of Philosophy in Biomedical Sciences Examination Committee Major Advisor: Robert Blumenthal, Ph.D. Academic Randall Worth, Ph.D. Advisory Committee: Sonia Najjar, Ph.D. Eric Lafontaine, Ph.D. Kevin Pan, Ph.D. Senior Associate Dean College of Graduate Studies Michael S. Bisesi, Ph.D. Date of Defense: April 18, 2007 Translational Control in Escherichia coli: Hfq and PvuII Doctor of Philosophy Meenakshi Kaul Kaw The University of Toledo College of Medicine 2007 DEDICATION To my parents, Dr. H. K. and Susheela Kaul, your prayers, love and constant encouragement has given me strength to turn this hardest personal challenge into reality. To my husband, Dr. Dinkar Kaw, for your love and unwavering support which has always been the most motivating forces in my life. ii ACKNOWLEDGEMENTS I am grateful for my major advisor, Dr. Robert Blumenthal for giving me his support, and opportunity of working in his laboratory. His close guidance, freedom, trust and respect over the years has been priceless. I also thank you for you for the scientific advice, as well as proffesional and personal advice in tough times. I would also like thank my previous advisor, Dr. Darren Sledjeski for his support, time, patience and scientific experties that helped to attain my goals. I would aslo like to thank my committee members for their invaluable time and patience and scientific advice they shared from time to time: Dr. Sonia Najjar, Dr. Eric Lafontaine, Dr. Randal Worth and my unofficial member Dr. Mark Wooten. I thank the State of Ohio and The University of Toledo for the pre-doctoral fellowship and the tution support provided to me for four and a half years of my study. I also thank the NIH for the Hfq project support. The material for PvuII project is based upon the work supported by NSF under Grant No. 0516692 and under the guidance of Dr. R.M. Blumenthal. iii I would also like to thank all the laboratory and Club Coli members, past and present, who have contributed to helpful scientific discussions. To name just few, Robert Lintner, John Lazarus, Iwona Mruk and Pankaj Mishra. Additionally I would like to thank the members of Department of Medical Microbiology and Immunology, especially Joyce Rodebaugh, Sharon Ellard, Sue Payne and Diane Ammons. I would like to thank my beautiful daughter Meesha Kaw for her immense support and patience, my mother inlaw Dr. Durga Kaw, my sisters Er. Kamakshi Kaul and Dr. Vinay Kaul. I would also like to thank my nephews Akash and Smish, nieces Arshee and Anapurna. Thank you for your love for me. Last but not least I would like to thank my cousin Er. Savita Bhan Wakhloo for her constant love and support. iv TABLE OF CONTENTS Page Dedication …………………………………....................... ii Acknowledgements …………………………………………………. iii Table of contents …………………………………………………. v Introduction …………………………………………………. 1 Literature …………………………………………………. 5 Manuscript I …………………………………………………. 40 Manuscript II ………………………………………………….. 90 Discussion ………………………………………………….. 143 Bibliography ………………………………………………….. 149 Abstract ………………………………………………….. 186 v INTRODUCTION In prokaryotic world, the majority of gene regulation appears to occur at the level of transcript initiation (Boelens and Gualerzi, 2002), in part because transcription and translation are concurrent. Neverthless the fastest way for the repertoire of cell proteins adapt to change in the environment or the cellular states is by regulating the translation. Translation can be repressed or enhanced by displacement, entrapment or competition (Schlax et al., 2001). During conditions of stress in the form of changes in pH, temperature, nutrient availbility, or osmolarity, cells often go into stationary phase (Loewen et al., 1998; Hengge- Aromis 1996). Various global regulators control the gene expression by different mechanisms that help the cell adapt to the unfavorable environment, and escape death. Hfq protein from Escherichia coli is a global regulator central to multiple regulatory processes (Muffler, et al., 1997; Tsui, et al., 1994). It is a key player in post-transcriptional regulation that acts by facilitating base-pairing of bacterial noncoding RNAs (ncRNAs) to their mRNA targets sites. (Schuppli, et al., 1997; Muffler, et al., 1997; Tsui, et al., 1997; Wassarman, et al., 2001). Evidence suggests that Hfq acts as a chaperone and modulates RNA structure (Moll, et al., 2003). Studies by Lease and Woodson, (2004) and E. Espinosa, P. Mikulecky and A. Feig, (personal communication) demonstrated that Hfq binds DsrA and rpoS RNAs independently and form stable ternary complexes. DsrA is a small 85 1 nucleotide ncRNA that is produced in response to low temperature and regulates translation of two global regulatory proteins, RpoS and HNS (Sledjeski and Gottesman, 1995) while rpoS mRNA specifies an alternative RNA polymerase sigma factor that plays a major role in transcribing stationary phase-associated genes (Pratt and Silhavy, 1998; Hengge-Aronis, 1996; Bougdour et al., 2004). The existing data imply that more than one site is required for Hfq to promote base-pairing between ncRNAs and their targets. We addressed these questions by mutational analysis of Hfq. My experiments in this study were designed for in vivo mutationally altered Hfq. I used reporter assays and RNA life time experiments. Combining these results with in vitro analyses by our collaborators at Indiana University, we hypothesized that there are at least two independent sites on the Hfq hexamer, and close proximity of these RNA-bound sites facilitate base-paring between the bound RNAs. In addition to the role of a global regulator such as Hfq, translational control can occur via cis-acting gene specific signals. I have studied this in genes for which regulation is particularly critical. Restriction Modification (RM) systems have a wide distribution in bacteria and archaea. RM systems include two components, a restriction endonuclease (REase) and a methyltransferase (MTase). I studied RM systems in which the REase and MTase are independent proteins (Hedgpeth, et al., 1972; Schildkraut I et al., 1984 Roberts, et al., 2003). 2 A subset of the type II RM systems include a third protein designated as controller or C protein that plays a regulatory role. PvuII is a C- dependent type II RM system derived from the Gram negative rod Proteus vulgaris (Gingeras et al, 1981) that was cloned and can be studied in E. coli (Blumenthal, et al., 1985). When RM systems move into a new host cell, it is crucial that the new host’s DNA be methylated protectively before REase expression occurs. The C proteins, such as C.PvuII play a central role in mediating this delay, and pre- expressing C.PvuII in a cell prevents transformation by the intact RM system (presumably due to premature REase production; (Vijesurier et al., 2000) Previous studies from our laboratory (Tao et al 1991; Tao et al., 1992; Vijesurier et al., 2000; Knowle et al., 2005) demonstrated the transcriptional regulation of REase by the C.PvuII. However, work from other laboratories also indicated that translational control by C protein can occur. A study of eco72IC demonstrated that C protein translationally controls expression and act as an activator of REase gene expression (Rimiseliene, et al., 1995) while C protein from the Esp13961 also demonstrated that it activates its own expression and its downstream REase gene by mechanism of translational coupling (Cesnaviciene, et al., 2003). Work from our laboratory indicated that C protein regulates REase expression via a transcriptional mechanism, but it is not clear whether this is sufficient to protect the cell. We investigated if there is some type of additional 3 translational-level control. Since there is an overlap region in this subset of C- controlled RM systems, we investigated whether this implied to some kind of translation control. In the PvuII RM system, there are two characteristics suggestive of translation regulation. First in PvuII and most C-dependent RM systems there is an overlap or close proximity between the C gene and the downstream R gene. Second in PvuII RM system this overlap is preceded by potential hairpins, one of which would occlude the ribosomal binding site (RBS) The set of experiments designed to analyze this study included cloning, site- directed mutagenesis, and beta-galactosidase assays. 4 LITERATURE REVIEW Part I: Translational control of genes in E. coli. Part II: Hfq as a regulator of translation mediated by DsrA RNA in E. coli Part III: C.PvuII as a regulator of translation of PvuII RM system in E. coli 5 PART I Brief history of translation The history of the evolution of the translationary machinery seems to have started with resolving the mysteries behind the evolutionary transition from RNA to DNA. The “RNA world” (Gilbert 1986) hypothesized that RNA was the major biological catalyst and genetic material before protein and DNA (Woes 1967; Crick 1968; Orgel 1968). The prediction from the RNA world model was that in the RNA world, protein synthesis evolved from RNA (Poole et al., 1998; Noller, 2004) before the three domains-namely archaea, bacteria, and eukaryotes separated out (Poole and Logan 2005). Futher research findings supported the evolution of protein from RNA and are accepted world wide. It is assumed that during the evolution of enzymatic
Details
-
File Typepdf
-
Upload Time-
-
Content LanguagesEnglish
-
Upload UserAnonymous/Not logged-in
-
File Pages192 Page
-
File Size-