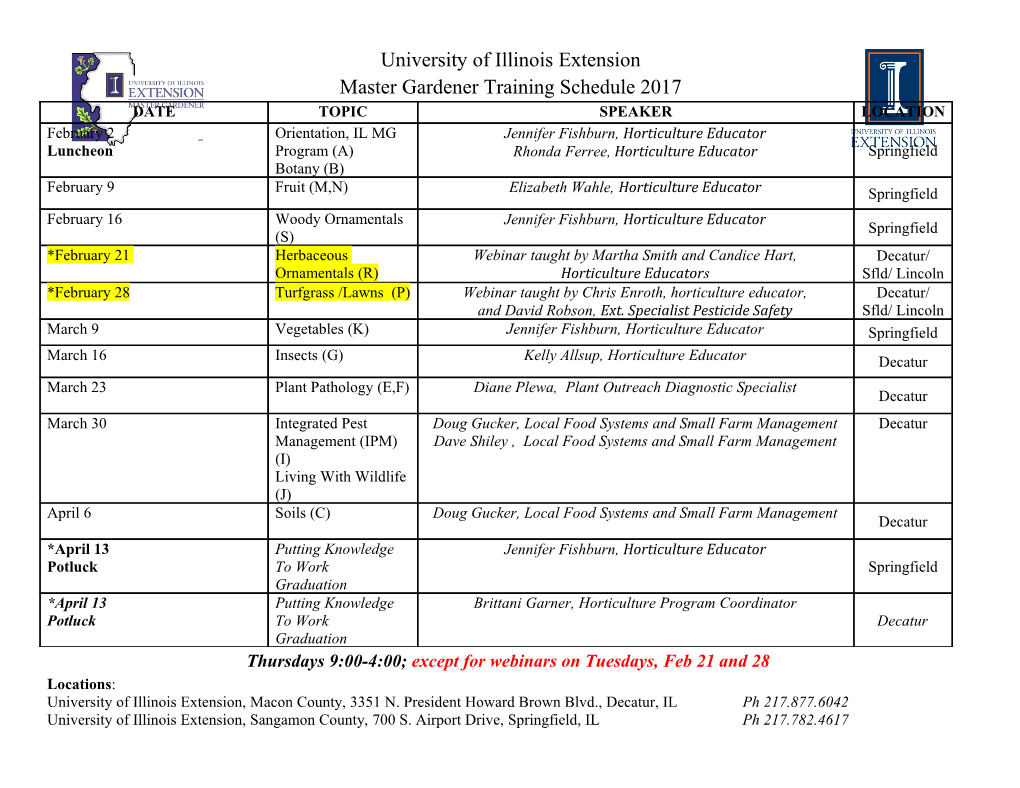
POTENTIAL FOR ENHANCING BIOCOMPATIBILITY THROUGH MICROSTRUCTURING AND ANTI-COAGULATING BIOMOLECULAR COATINGS by TINA TALWAR (Under the Direction of William Kisaalita) ABSTRACT To demonstrate the potential for increasing implantable sensor life span by sensor surface microstructuring, microwells were fabricated into SU-8(an epoxy based negative photoresist material). The microstructured surfaces were modified with heparinized medical grade polyurethane and the biocompatibility of the surfaces was evaluated with respect to protein adsorption and porcine platelet adhesion. With a commercial implantable sensor surface (MINIMED), as a control, the microstructured/ heparinized surfaces offered more resistance (p=0.05) to protein and cell attachment, suggesting potential for increasing implantable sensor lifespans through microstructuring. INDEX WORDS: Biofouling, Biosensor, Cell-material interaction, Microstructuring, SU-8 POTENTIAL FOR ENHANCING BIOCOMPATIBILITY THROUGH MICROSTRUCTURING AND ANTI-COAGULATING BIOMOLECULAR COATINGS by Tina Talwar B.E., Dr. Babasaheb University, India, 1998 A Thesis Submitted to the Graduate Faculty of The University of Georgia in Partial Fulfillment of the Requirements for the Degree MASTER OF SCIENCE ATHENS, GEORGIA 2012 © 2012 Tina Talwar All Rights Reserved POTENTIAL FOR ENHANCING BIOCOMPATIBILITY THROUGH MICROSTRUCTURING AND ANTI-COAGULATING BIOMOLECULAR COATINGS by TINA TALWAR Major Professor: William S. Kisaalita Committee: Yiping Zhao William Tollner Electronic Version Approved: Maureen Grasso Dean of the Graduate School The University of Georgia May 2012 ACKNOWLEDGEMENTS I express gratitude to Dr. William Kisaalita, Dr. Robert Brown, Ju Rong and the Department of Veterinary Science for their support in my academic career. iv TABLE OF CONTENTS CHAPTER 1: Implantable Glucose Sensors................................................................................... 1 1. 1 Introduction .......................................................................................................................... 1 1.2 Objectives ............................................................................................................................. 3 References ................................................................................................................................... 4 CHAPTER 2: Biocompatible Polymers and Anticoagulants .......................................................... 6 2. 1 Literature Review................................................................................................................. 6 2.2 Biocompatible Polymers - Advantages and Disadvantages .................................................. 9 2.3 Heparin and PU Mechanism ............................................................................................... 12 2.4 Anticoagulant ...................................................................................................................... 12 2.5 PU Synthesis ....................................................................................................................... 13 References ................................................................................................................................. 14 CHAPTER 3: Polymer Selection with Quartz Crystal Microbalance (QCM) ............................. 18 3.1 Abstract ............................................................................................................................... 18 3.2 Introduction ......................................................................................................................... 19 3.3 Polymers evaluated ............................................................................................................. 20 3.4 Polymer selection ................................................................................................................ 22 References ................................................................................................................................. 25 CHAPTER 4: Potential for enhancing Biocompatibility through microstructuring and anti- coagulating biomolecular coating ................................................................................................. 27 4.1 Abstract ............................................................................................................................... 28 4.2 Introduction ......................................................................................................................... 29 4.3 Materials and Methods ........................................................................................................ 30 4.4 SEM and X-ray analysis ..................................................................................................... 34 4.5 Results and Discussion ....................................................................................................... 34 4.6 Concluding remarks ............................................................................................................ 37 References ................................................................................................................................. 38 Acknowledgements ................................................................................................................... 42 CHAPTER 5: Conclusion and Future Directions ......................................................................... 48 5.1 Discussion of results ........................................................................................................... 48 5.2 Future Directions ................................................................................................................ 49 APPENDIX 1: PU synthesis and modification with pendant acetylated thiol groups ................. 51 APPENDIX 2: PU and heparin binding........................................................................................ 53 APPENDIX 3: SU-8 Coating and Micro patterning ..................................................................... 55 APPENDIX 4: PPP, PRP extraction and Cell Counting .............................................................. 60 APPENDIX 5: Cell Adhesion on SU-8 micro wells .................................................................... 62 APPENDIX 6: PU spin coating parameters.................................................................................. 63 v CHAPTER 1: Implantable Glucose Sensors 1. 1 Introduction The design of in vivo glucose biosensors for clinical purpose remains a significant challenge mostly due to poor biocompatibility. When a sensor comes into contact with blood, it provokes a defensive reaction of the blood. The human body regards any sensor implanted either subcutaneously or placed in the blood as foreign and tends to reject it by fouling the sensor surface. Implantable sensors are fouled by protein adsorption followed by platelet adhesion that change the cells‟ morphology and activate them; leading to formation of thrombus [1]. Thrombin effectuates conversion of fibrinogen into fibrin, an insoluble mass of strands. The activated platelets and fibrin form thrombus emboli. When these thrombi detach from the sensor it causes infection and form a biofilm or a scar tissue. This fouling of sensor negatively impacts the long- term utility of the sensor by reducing glucose diffusion to the sensor which results in decreased current output. This leads to either partial or complete malfunction of the sensor calling for frequent replacement, i. e. every 2-7 days [2, 3]. Although, reliable sensor performance in vitro has been reported, a continuous glucose monitoring in vivo is still in experimental stages. In all the studies mentioned here sensor performance has been achieved mainly by polymer coatings such as polyurethane and anti coagulants such as heparin. Amperometric sensors based on glucose oxidase have come a long way since their introduction in 1962 by Clark and Lyons. In 1995, needle type sensors with a „long‟ life span of 25 days was reported. The longest lifespan 1 of an implantable electrochemical type of glucose sensors under clinical trials is about 4 -7 days only and in vitro stability stretching to about 56 days. The long term stability of such polyurethanes (PU)-epoxy in bovine serum is reported to be 6 months [4]. In 2000, Yang et al., reported a PU as a diffusion limiting membrane with stable output in bovine serum for 70 h and linearity up to 50 mM. Another study reported the use of bulk and surface heparinization with in vivo efficacy ranging from a week to a few months [5]. Polyurethanes (PU) containing Ag have shown lowered foreign body reaction for up to 19 days [6], which is still not sufficient for long term usage in vivo. Another factor compromising life of the sensor is a decline in enzyme activity over time. For long-term application, a fully implanted glucose sensor that works reliably for a month to at least six months is desirable. A possible approach in increasing the lifespan of the sensors may be achieved by coating them with anti-biofouling and anti- coagulating materials [7] and providing a constant supply of enzyme [8]. All the above mentioned studies have tackled either protein or cell attachments issues separately, demonstrating potential for increasing in vivo life of sensors, but have not achieved the desired long life span of the sensor yet. The present study to explore increasing the life span of amperometric type glucose sensors is based
Details
-
File Typepdf
-
Upload Time-
-
Content LanguagesEnglish
-
Upload UserAnonymous/Not logged-in
-
File Pages73 Page
-
File Size-