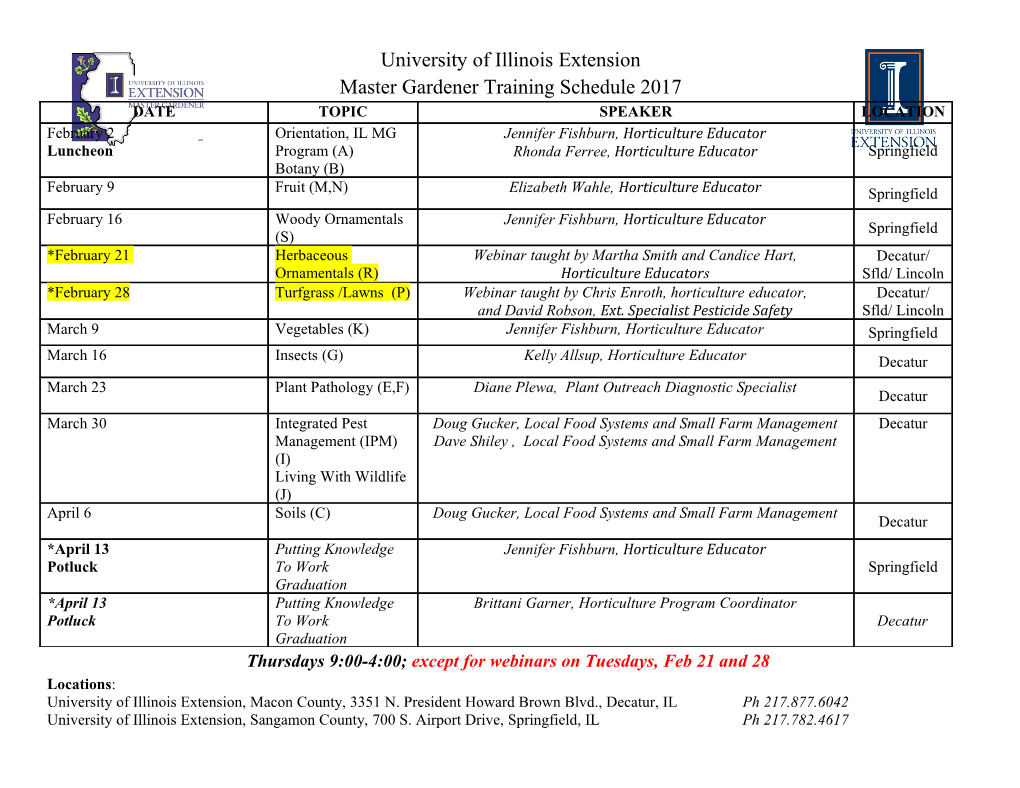
Pergamon Int. 1. Irzsecr Morphol. & EmbryoI., Vol. 25, No. l/2, pp. 6341, 19% Copyright @I 1996 Elsevier Science Ltd Printed in Great Britain. All rights reserved 0020-732Z% $15.00 + 0.00 OOZO-7322(95)00023-2 POLYTENE CHROMOSOMES: A GENERAL MODEL FOR THE EUCARYOTIC INTERPHASE STATE Horst Kress Institut fur Genetik, Freie Universitat Berlin, Arnimallee 7, 14 195 Berlin, Germany (Accepted 7 November 1995) Abstract-The euchromatic structures of insect polytene chromosomes represent an amplified version of the chromomeric organization in diploid chromatin. As such, they are an excellent model system for studying structure/function relationships of eucaryotic interphase chromosomes. Polytenization is accomplished by replication patterns that are different for euchromatic and heterochromatic chromatin and also seem to depend on the functional state of chromatin. In Diptera, polytene chromosomes are characterized by genetically determined discontinuities of DNA packing in bands and interbands that are modified by functional demands. The cytological visualization of proteins that are associated with the compaction and decondensation of chromatin, allows the analysis of the temporal and spatial dynamics of DNA/protein interactions in the context of structure, transcription, and the processing of RNA. Ribonucleoprotein particles may be followed on their way via the nuclear matrix through the pores of the nuclear envelope to the cytoplasmic compartment. Thus, polytene nuclei provide unique opportunities for studying the flow of genetic information from the site of storage to the site of action, i.e. from gene to phenotype. Copyright 0 1996 Elsevier Science Ltd. Index descriptors (in addition to those in title): Chromatin, replication, transcription, gene expression, RNA processing, RNA transport. Genetics without an exact knowledge of the eucaryotic chromosome organization is like physics in the time of Maxwell-without a knowledge of the structure and organization of the atom. Lima-de-Faria (1983) 1. INTRODUCTION The presence of polytene chromosomes has been described in a limited number of plants and animals. They are generally found in cells or organs that are engaged in secretory functions, or those that nourish or support the development and differentiation of cells or embryos, i.e. are usually characterized by high rates of RNA and protein synthesis (Table 1). Polytene chromosomes, since their discovery as a cytogenetic tool in Drosophila about 60 years ago, have become important in solving major problems in special fields of biological research. Beermann’s (1952) classical investigations on Chironomus salivary gland chromosomes focused attention on structure/function relationships of gene expression at the chromosome level. He concluded that the structural modifications known as puffs are the consequence of differential gene activity, a view that was supported by the visualization of RNA synthesis at such sites (Pelling, 1955). Becker (1959, 1962) demonstrated that in Drosophila, the ontogenetic changes in puff formation and regression, occurring during the larval/prepupal transition, must 63 64 H. Kress Table 1. Occurrence of polytene chromosomes in plants and animals* Species+ Tissue Level of polyteny* Plants Monocotyls Scilla bifolia (Lil.1) Antipodal cells 1024C Clivia miniata (Lil.) Antipodal cells 128n Allium ursinum (Lil.) Endosperm haustoria Dicoryls Aconitum ranunculifolium (Ran.) Antipodal cells 128n Papaver rhoeas (Pap.) Antipodal cells 128n Phaseolus coccineus (Fab.) Suspensor 8192n Tropaeolum majus (Ger.) Suspensor 2048C Rhinanthus alectorolophus (Ser.) Endosperm haustoria 384n Thesium alpinum @cr.) Endosperm haustoria 384n Bryonica dioica (Cut.) Anther hairs 256C Ciliata Stylonychia mytilus (Spi.) Macronucleus anlage 4096C” (transiotory) lnsecta Bilobella massoudi (Co].) Salivery gland Schistocerca gregariaJ3al.) Fat body (enocytes) Bombyx mori (Lep.) Silk gland 524,288C Calliphora erythrocephala (Dip.) Trichogen cells 4096nn Chironomus tentans (Dip.) Salivary gland 32,768n Chironomus thummi (Dip.) Salivary glands 8192C Malpighian tubules 16,394C Sarcophaga bullata (Dip.) Foot pads 2,048C Drosophila melanogaster (Dip.) Salivary gland 1024n; 2048C Mammalia Micro&s arvalis (Rod.) Trophoblast 2048C Mus musculus (Rod.) Trohoblast 512-1024C Rattus rattus (Rod.) Trophoblast 1024C; 4096C *Selected from Nag1 (1978, 1981). ‘Cal. = Collembola; Cut. = Cucurbitales; Dip. = Diptera; Fab. = Fabales; Ger. = Geraniales; Lep. = Lepidoptera; Lil. = Liliales; Pap. = Papaverales; Ran. = Ranunculales; Rod. = Rodentia; Sal. = Saltatoria; Ser. = Scrophulariales; Spi. = Spirotrichia. *C values refer to DNA measurements, n values to other methods (chromosome counts, volumetry). OAmmermann et al. (1974). s = Ribbert (1967). be controlled by a humoral factor. It was shown in Chironomus to be the moulting hormone (ecdysone) (Clever and Karlson, 1960). These investigations were of general interest, because they provided the basis for a new concept of steroid hormone action at the chromosome level (Karlson, 1963). Later, the phenomenon of sequential gene activation was discovered (Clever, 1964), and subsequently analyzed in detail in Drosophila by Ashburner and his associates, who finally proposed a formal model of interactions between the ecdysone-receptor complex and early and late target sites (Ashburner et al., 1974). In addition to ecdysone-induced puffing, the formation of puffs by heat shock turned out to be a second gene-expression system of general importance. Originally described in the polytene chromosomes of Drosophila (Ritossa, 1962), the heat-shock response Polytene Chromosomes: Eucaryotic Interphase State Model 65 has been found in a wide variety of pro- and eucaryotes, and is now regarded as a general protection mechanism in living cells against damage caused by stress conditions. The discovery that the expression of several of the heat-shock genes is also controlled by ecdysone (Ireland and Berger, 1982) provided a formal basis for connecting the hormonal regulatory networks of development with those of the maintenance of cell function in the context of thermotolerance. The cloning of ecdysone- and heat-shock-induced genes for elucidating the molecular mechanisms of converting external signals into specific patterns of gene expression, was a logical consequence of these basic cytological investigations. However, for the comprehensive understanding of the orchestration of gene expression, the analyses had to be extended to the nuclear compartment too. The experimental approach to this aspect of gene expression may be traced back to early attempts to isolate RNA from Chironomus Balbiani Rings (gigantic puffs), which subsequently became an impor- tant model system for studying the structure of active genes, the formation of ribonucleoprotein (RNP) particles, their transport through the nuclear matrix, and the translocation of transcripts across the nuclear membrane (reviewed by Daneholt, 1974; Mehlin and Daneholt, 1993). During the last decade, a multitude of non-isotopic methods for the detection of nucleic acid and protein targets became available. The application of these methods in whole-mount preparations of nuclei and cells in combination with new microscopical methods (e.g. confocal microscopy) opened a new exciting field of the spatial visualization of events related to gene expression. Although, in that way, the cytological analysis of interphase chromatin and the nuclear matrix has also become possible in diploid cells, polytene nuclei offer the unique opportunity of correlating reporter signals and visible chromosomal structures. In addition, the larger size of these nuclei provides a better spatial resolution of perichromosomaYinterchromosoma1 relationships. This article describes the present view of polytene chromosome structure, their replicative and transcriptional activities, and aspects of nuclear RNA transport. Owing to space limitations, it will focus only on more recent developments. For information on earlier literature, the reader should consult reviews by Alfert (1955), Beermann (1962, 1972), Berendes (1973), Ashburner (1970, 1980), Daneholt (1974, 1975), Nag1 (1978, 1981), Zegarelli-Schmidt and Goodman (1981), Zhimulev et al. (1981), Richards (1985), Hill and Rudkin (1987), Korge (1987), Sorsa (1988) and Hofmann and Korge (1993). 2. ACQUISITION OF POLYTENY DURING DEVELOPMENT Polytenization of chromosomes is accomplished by “endo-cycles”, which are defined as replication cycles within the nuclear envelope without spindle formation (Geitler, 1953; Nagl, 1978). In such cells, the cell cycle is reduced to an S- and a G-phase without karyo- and cytokinesis. In dipterans, most of the larval cells enter the endo-cycle pathway, and only cells destined to become precursors of imaginal cells and of nerve cells maintain diploidy. We are just beginning to understand the underlying control mechanisms for switching cells from the mitotic cell cycle to its somatic dead-end short-circuit version. In Drosophila melanogusfer, it is now well established that a Gl phase is acquired for the first time in the 17th embryonic cell cycle. During its prolonged duration, a decision is made between continuing mitotic cycles or entering endo-cycles 66 H. Kress (Edgar and O’Farrell, 1990). Endoreplication commences at cycle 18 in different regional domains of the embryo, and is later on restricted to distinct periods (Smith and Orr-Weaver, 1991). We have to assume that the genes involved in determining the endo-cycle pathway must
Details
-
File Typepdf
-
Upload Time-
-
Content LanguagesEnglish
-
Upload UserAnonymous/Not logged-in
-
File Pages29 Page
-
File Size-