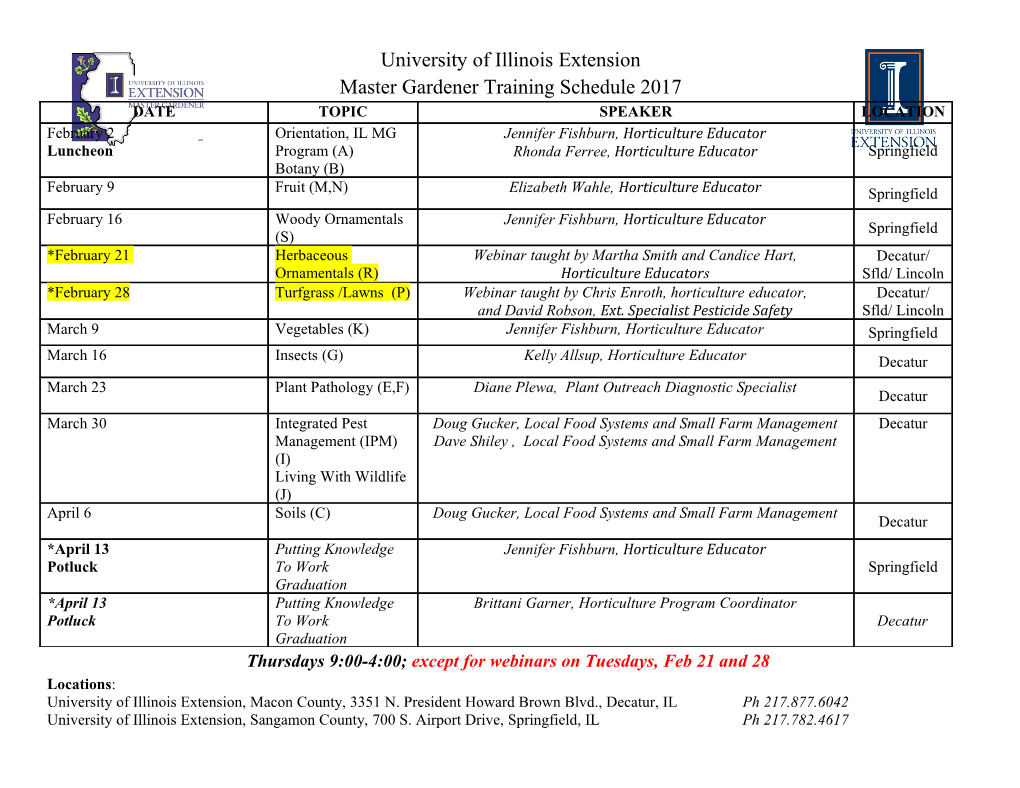
doi: 10.1111/j.1365-3121.2007.00737.x On subducting slab entrainment of buoyant asthenosphere J. Phipps Morgan,1 Jo¨ rg Hasenclever,2,3 M. Hort,3 L. Ru¨ pke2,4 and E. M. Parmentier5 1Department of Earth and Atmospheric Sciences, Cornell University, Ithaca, NY, USA; 2IfM-GEOMAR, Kiel University, Kiel, Germany; 3Institute of Geophysics, Hamburg University, Hamburg, Germany; 4Department of Physics, PGP, Oslo University, Oslo, Norway; 5Department of Geological Sciences, Brown University, Providence, RI, USA ABSTRACT Laboratory and numerical experiments and boundary layer upon the subduction rate and the density contrast and viscosity analysis of the entrainment of buoyant asthenosphere by of the asthenosphere, while the upper (cold) side of the slab subducting oceanic lithosphere implies that slab entrainment is may entrain as much by thermal ÔfreezingÕ onto the slab as by likely to be relatively inefficient at removing a buoyant and mechanical downdragging. This analysis also implies that lower viscosity asthenosphere layer. Asthenosphere would proper treatment of slab entrainment in future numerical instead be mostly removed by accretion into and eventual mantle flow experiments will require the resolution of 10– subduction of the overlying oceanic lithosphere. The lower (hot) 30 km-thick entrainment boundary layers. side of a subducting slab entrains by the formation of a 10– 30 km-thick downdragged layer, whose thickness depends Terra Nova, 19, 167–173, 2007 the Basin and Range (Goes and van 1975; Karato and Wu, 1993) than it Introduction der Lee, 2002) in the western U.S. does as cooler temperatures. As the Typical cartoons of mantle convection However, beneath stable continental uppermost suboceanic mantle is clo- show oceanic lithosphere moving in regions such as the Archaean shields sest to its solidus temperature, the concert with the underlying mantle in W. Australia (Gaherty et al., 1999) asthenosphere could simply be a con- flow (Fig. 1a). If, however, the and N. America (Goes and van der sequence of this effect, and the pres- asthenosphere layer – presumed to Lee, 2002) seismic wavespeeds are ence of any partial melt would underlie at least much of the oceanic significantly faster, implying the pres- enhance the reduction in viscosity lithosphere – is itself less dense and ence of much stronger (lithospheric) (Hirth and Kohlstedt, 1995) and seis- viscous than the underlying mantle, mantle between 60 and 250 km depths mic wavespeeds (Hammond and then the resulting asthenosphere cir- (see also Gung et al., 2003). The LVZ Humphreys, 2000). A higher water culation can be strongly altered in is an observed fact. Most researchers content has also been suggested to be ways that may have significant geolo- believe the LVZ also corresponds to a the origin for the LVZ (Karato and gical consequences (Fig. 1b,c). Here, lower viscosity region within EarthÕs Jung, 1998), and to lead to a viscosity we study the question of how effi- mantle. Rock-mechanics arguments, reduction in this region of the mantle ciently a subducting slab will entrain for example, imply the existence of a (cf. Hirth and Kohlstedt, 1996). buoyant asthenosphere near a subduc- low-viscosity zone at the shallowest We favour the hypothesis that tion zone, a question that leads to a hot mantle where competing tempera- EarthÕs asthenosphere forms as a sim- better understanding of the behaviour ture and pressure effects lead to a ple consequence of mantle plume up- of the asthenosphere–lithosphere sys- viscosity minimum (Weertman and welling – as hot buoyant plume tem in mantle convection. Weertman, 1975; Buck and Parmen- material naturally tends to rise, up- There exist several different views tier, 1986; Karato and Wu, 1993). welling plumes bring hot buoyant on what exactly is asthenosphere. Studies of the distribution of stresses in material to the ÔceilingÕ of the con- Seismic observations of the radial the oceanic plates require a low vis- vecting mantle that is formed by the Earth structure show a low-seismic- cosity asthenosphere of 1018–1019 Pa-s base of the mechanical lithosphere velocity (Dziewonski and Anderson, underneath the ocean lithosphere (Phipps Morgan et al., 1995a). In this 1981) and high attenuation (Widmer (Richter and McKenzie, 1978; Wiens case, asthenosphere material will actu- et al., 1991) layer between 100 and and Stein, 1985), consistent with the ally be hotter than the underlying 300 km depths. This layer with low 1018–1019 Pa s viscosities inferred mantle (at least in terms of its poten- seismic wavespeeds is now known to from post-glacial rebound at Iceland tial temperature; e.g. temperature cor- be well developed beneath the 60– (Sigmundsson and Einarsson, 1992). rected for adiabatic effects). If the 100 km-thick oceanic lithosphere Several potential mechanisms have asthenosphereÕs average temperature (Gaherty et al., 1999) and active been suggested to cause the formation is that of hot upwelling plumes, then young continental margins such as of a shallow asthenosphere layer with an observation of the deflection of the low wavespeeds, high attenuation and 410- and 660-km discontinuities be- Correspondence: J. Phipps Morgan, reduced viscosity. Silicate mantle close neath Iceland suggests that it may be Department of Earth and Atmospheric to its melting temperature will have 10–20% (i.e. 150–300°C) warmer Sciences, Cornell University, Ithaca, NY slower wavespeeds, higher attenuation than the underlying ambient mantle USA. Tel.: +1 607-821-1741; fax: +1 607- (Faul and Jackson, 2005), and lower (Shen et al., 1996). This would result 254-4780; e-mail: [email protected] viscosity (Weertman and Weertman, in a 0.5–1% reduction in density of Ó 2007 Blackwell Publishing Ltd 167 On subducting slab entrainment of buoyant asthenosphere • J. Phipps Morgan et al. Terra Nova, Vol 19, No. 3, 167–173 ............................................................................................................................................................. (a) viscous deformation mechanism in the uppermost mantle, dislocation creep of olivine at 1600 K, a 200 K increase in temperature leads to a >100-fold viscosity reduction. Numerical and laboratory experiments of asthenosphere (b) slab-entrainment We first discuss several numerical experiments that explore the entrain- ment surrounding a subducting slab. The key question we are interested in is how much asthenospheric material is downdragged by subducting slabs. (c) Focusing on the effects of subduction processes, we also exclude mantle plumes as part of the numerical mod- elling domain. In order to highlight the implications of a hot and depleted, hence weak and buoyant astheno- sphere layer we have simplified our setup for the numerical calculations in that phase transitions and melting pro- cesses are not considered. Instead, we begin with a preexisting 200 km-thick asthenosphere layer with a higher tem- perature and more depletion than the underlying mantle and ask how quickly Fig. 1 (a) Cartoon illustrating the ÔtypicalÕ connection of lithospheric plate motions with deeper mantle flow. (b) Cartoon illustrating the possible connection between plate it can be removed by plate subduction. motions and deeper flow when a buoyant low-viscosity asthenosphere decoupling Consider the buoyant astheno- zone is present. (c) A snapshot after 7 Ma from a numerical experiment discussed in sphere entrainment scenario sketched the text that shows the behaviour illustrated in (b). Here the white line marks the in Fig. 1(b) and Box 1. Intuition sug- boundary of asthenosphere material – a gradational asthenosphere–mesosphere gests a simple flow pattern may form, transition (centred around 300 km depth) was made by having the asthensosphere– and this pattern is in fact seen in the mesosphere interface conductively cool for 50 Ma in a static configuration before numerical and laboratory experi- surface plate motions were Ôturned onÕ. The oceanic lithosphere and slab are assumed ments. The moving plate tends to to move at 63 km Ma)1. White streamlines in the mantle wedge show the recirculation drag shallower asthenosphere towards that develops in this region, colours show asthenosphere and mesosphere speeds, and the subduction zone, which creates a arrows show instantaneous flow directions. (The experiments with a gradational relative pressure high that, in turn, asthenosphere–mantle interface evolve quite similarly to previous experiments in locally tilts the asthenosphere base which this transition was assumed to be discontinuous, as seen by comparing the and drives deeper lateral astheno- evolution of supplementary videos oceanside.mpg and oceanside_sharp.mpg.) The sphere flow away from the subduction asthenosphere return flow beneath the base of the oceanic lithosphere is in good zone as discussed in previous studies agreement with Schubert and TurcotteÕs (1972) simple boundary layer model, a (Schubert and Turcotte, 1972; Phipps boundary layer theory for the entrainment around the slab is discussed in Box 1. Morgan and Smith, 1992). In addi- tion, the subducting slab tends to drag the asthenosphere in comparison with larger than the decrease in thermal thin sheets of asthenosphere into the the underlying cooler mantle (Dq ⁄q = buoyancy due to cooling associated deeper mantle at its top and bottom ) ) aDT, with a 3.3 · 10 5 °C 1). Any with the latent heat of partial melting boundaries (Fig. 1b,c). All of these partial melt extraction from upwelling (cf. Phipps Morgan et al., 1995b). Dep- effects are clearly visible in our numer-
Details
-
File Typepdf
-
Upload Time-
-
Content LanguagesEnglish
-
Upload UserAnonymous/Not logged-in
-
File Pages7 Page
-
File Size-