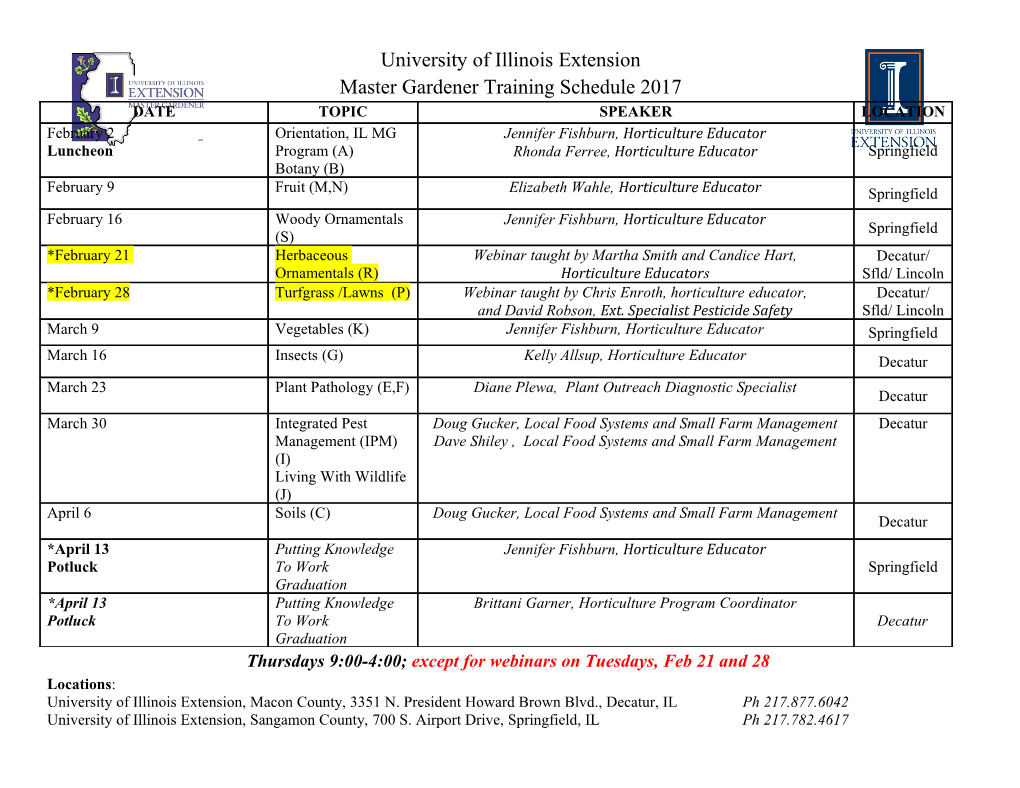
VOLUME 14 JOURNAL OF CLIMATE 1MARCH 2001 LETTERS On the Relationship between Tropical Convection and Sea Surface Temperature ADRIAN M. TOMPKINS* Max-Planck-Institut fuÈr Meteorologie, Hamburg, Germany 9 August 2000 and 12 September 2000 ABSTRACT Tropical observations show convective activity increasing sharply above sea surface temperatures (SSTs) of around 268C and then decreasing as the SST exceeds 308C, with maximum observed SSTs of around 328C. Although some aspects of this relationship are reasonably well understood, as of yet no theory has explained the decrease in convective activity above 308C. Here it is suggested that this aspect of the relationship could result from a organizational positive feedback, sometimes termed ``self aggregation,'' whereby the occurrence of convection makes future convection more likely to occur in the same location. Using cloud-resolving simulations, it is shown that the feedback between convection and the water vapor ®eld is a good candidate for this role. Deep convection in the Tropics is intimately tied to ocean dynamics (Newell 1979; Ramanathan and Collins the general dynamical circulation. Thus to understand 1991; Fu et al. 1992; Wallace 1992; Clement et al. 1996; the observed relationship between convection and sea Sun and Liu 1996). All current thermostat theories in- surface temperature (SST) in the Tropics is also to gain volve convection. For example, Ramanathan and Collins a general understanding of tropical dynamics. The ob- (1991) proposed that cirrus clouds associated with con- servations show that above a ``threshold'' SST of 268C, vection regulate SST by the reduction of the surface convective activity increases sharply (Graham and Bar- incident shortwave (SW) radiation. Wallace (1992) nett 1987; Waliser and Graham 1993; Zhang 1993). By states that surface ¯uxes and the large-scale ¯ow will sorting the observations into classes of large-scale dy- control SSTs even in the absence of SW cloud forcing. namical activity (i.e., convergence or divergence re- However, convection is still invoked to ``vent'' the high gimes), Lau et al. (1997) and Bony et al. (1997) revealed SST area, and prevent the boundary layer (BL) and that this increase is due to the horizontal SST gradient, surface temperatures from warming in unison. The role and in fact convection is largely independent of the of convection via both enhanced surface ¯uxes and SW absolute value of the local SST. This has been con®rmed forcing has recently been emphasized (Sud et al. 1999). using cloud-resolving models (CRMs; Lau et al. 1994; Other theories involve convection in ocean mixed layer Tompkins and Craig 1999). Thus we can view convec- dynamics (Anderson et al. 1996) or as part of a coupled tive activity and the general tropical circulation as syn- large-scale circulation that forces the upwelling of cool onymous; an increase in convective activity must be waters (Clement et al. 1996; Sun and Liu 1996). concurrent with an increase in mean atmospheric ascent. This leads us to the third aspect of the convection± The observations also show that SST is limited to SST relationship, namely the reduction in convective about 328C, and the fact that temperatures in the ex- activity above SSTs of around 308C. As documented by tensive ``warm pool'' region of the western Paci®c are Waliser (1996), these warmest SSTs often occur within within one or two degrees of this maximum has led to the Paci®c warm pool region or the Indian Ocean, with the suggestion of a number of SST thermostat mecha- timescales of weeks to a few months, and are termed nisms (i.e., negative feedbacks), involving cloud radi- SST ``hot spots'' or ``warm anomalies.'' These regions ative forcing, surface latent ¯uxes, and atmospheric and of warmest SST are often free from convection. Thus the argument is that a lack of local convection (for what- ever reason) allows the SST to increase due to increased * Current af®liation: ECMWF, Reading, United Kingdom. solar insulation and reduced surface latent heat ¯uxes. The SST does not run away, however, since it is even- tually controlled by the thermostat mechanisms involv- Corresponding author address: Dr. A. M. Tompkins, ECMWF, Shin®eld Park, Reading RG2 9AX, United Kingdom. ing convection. E-mail: [email protected] However, as it stands this argument leads to an ap- q 2001 American Meteorological Society 633 Unauthenticated | Downloaded 09/29/21 06:41 PM UTC 634 JOURNAL OF CLIMATE VOLUME 14 parent paradox. If convection is generally increasing (1998) and references therein, while the experimental with SSTs above 268C, and SSTs are ef®ciently limited setup is discussed in more detail in Tompkins (2001). by convective thermostats, how can developing SST hot A uniform 2 K day21 cooling provides the forcing for spots remain free from convection for periods of weeks convection. An underlying SST gradient is imposed to months, allowing the SST anomaly to amplify? It is along the 1024-km axis, taking the form of one sine sometimes claimed (e.g., Waliser 1996; Lau and Sui wave with SSTs ranging from 299.5 to 300.5 K, giving 1997) that ``remotely forced'' atmospheric descent pro- SST gradients comparable to those observed along the vides the mechanism for convective suppression. This equatorial Paci®c. The SST gradient is imposed for a leads to a picture where a negative feedback thermostat period of 5 days, in order to establish convection over prevents runaway SSTs, with large-scale ¯ow variability the warmest SSTs. Over the cooler SSTs, convection shutting off convection, allowing hot spot development. should become suppressed by mean subsidence. Thus a But there is a problem with this view. To illustrate large-scale overturning circulation is established, mod- this, we use the example of an incipient SST hot spot. eled for the ®rst time in a 3D framework with resolved For current thermostat theories to work, the BL above deep convection. this warm patch must be reasonably closely related to Over the coolest SSTs, where deep convection is sup- the surface thermodynamically, although the water va- pressed, the increase in shortwave incoming ¯ux, and por difference between the surface and atmosphere is the reduction of surface latent heat ¯ux, would result likely to increase due to low mean winds in the sup- in a surface ¯ux imbalance. Within the warm pool and pressed region (Zhang et al. 1995). Thus under current Indian Ocean regions, this imbalance can cause SSTs theories, one would expect positive SST anomalies to to increase (e.g., Lau and Sui 1997; Woolnough et al. be accompanied by higher values of boundary layer 2000b), perhaps eventually creating a SST warm anom- equivalent potential energy (ue). The reason why this aly. We simulate the warm anomaly development in a high ue does not lead to deep convection, removing the very idealized manner, by simply reversing the SST SST perturbation on the timescale of a day or less, is gradient at day 5 of the experiment, to create a surrogate that remote forcing prevents this. This seems quite rea- hot spot. Although extremely idealized, this experiment sonable until we realize that we are deceiving ourselves allows us to examine the atmospheric dynamical re- with a subtle terminology change. What remote forcing sponse to SST anomalies, in an framework where both actually refers to is ``remote convection,'' since we have large- and convective-scale 3D circulations are explic- seen that convection and large-scale ¯ow are commen- itly represented. surate. Thus, the assertion that hot spots are the result Figure 1 shows the surface precipitation, averaged of remote forcing implicitly contains the assumption that across the 64-km axis, revealing the location of con- some, as yet undetermined, process ensures that regions vection during the experiment. After an initial period over remote cooler SSTs remain more favorable to deep of random convection, the large-scale circulation is es- convection over a period of time long enough to allow tablished during the ®rst 5 days, with ascending motion hot spot development. Either some unspeci®ed process over the warmest SSTs as expected. After the SST re- prevents the ue of BL air over the warmest SSTs from versal on day 5, the convection dies out quickly over exceeding that of remote cooler SST regions, or if this the cool SSTs. However, convection does not sponta- is not the case, the BL ue air over the warmest SSTs is neously ¯are up over the new SST hot spot, but instead somehow prevented from forming deep convection. propagates slowly toward it. Computing resources limit Convection over land, for example associated with the the experiment to ten days, but judging from the prop- Asian monsoon, can not be invoked as an alternative agation speeds, at least two weeks would be required remote forcing candidate, since there is no obvious rea- for the convection to reach the highest SSTs. son that convection over higher SSTs will be suppressed Closer examination of the thermodynamic ®elds re- in preference to lower SST areas. veals a possible reason for thisÐthe interaction with To shed light on this problem, an idealized experiment water vapor. Figure 2 shows how, even by day 10, the is conducted, using a 3D model with a 2-km horizontal region centered at x 5 768 km, that was free from resolution to resolve the dynamics of convection, and convection during the ®rst 5 days of the experiment, is that represents many ice and warm rain microphysical very dry. It is well known that convection locally moist- processes that occur in clouds. The model uses a domain ens its environment by detraining water vapor and cloud of 1024 km by 64 km in the horizontal, and 20 km deep, condensate. On the other hand, the subsidence drying with horizontal periodic boundary conditions.
Details
-
File Typepdf
-
Upload Time-
-
Content LanguagesEnglish
-
Upload UserAnonymous/Not logged-in
-
File Pages5 Page
-
File Size-