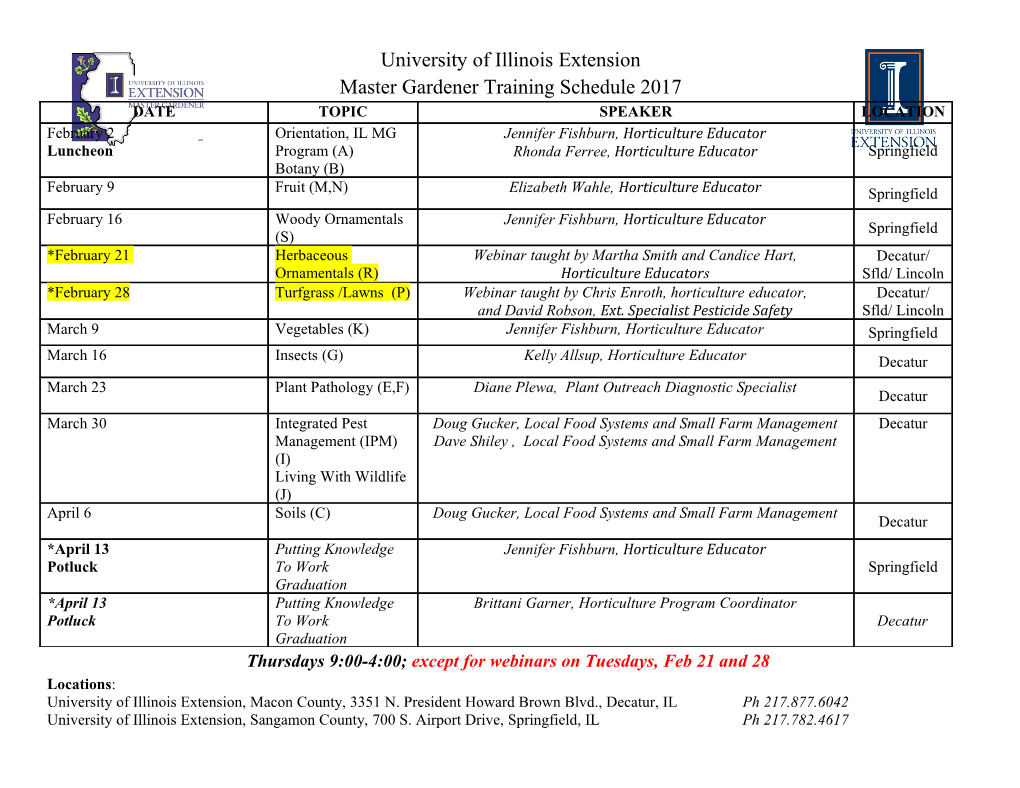
High Power Laser Science and Engineering, (2019), Vol. 7, e54, 54 pages. © The Author(s) 2019. This is an Open Access article, distributed under the terms of the Creative Commons Attribution licence (http://creativecommons.org/ licenses/by/4.0/), which permits unrestricted re-use, distribution, and reproduction in any medium, provided the original work is properly cited. doi:10.1017/hpl.2019.36 Petawatt and exawatt class lasers worldwide Colin N. Danson1;2;3, Constantin Haefner4;5;6, Jake Bromage7, Thomas Butcher8, Jean-Christophe F. Chanteloup9, Enam A. Chowdhury10, Almantas Galvanauskas11, Leonida A. Gizzi12, Joachim Hein13, David I. Hillier1;3, Nicholas W. Hopps1;3, Yoshiaki Kato14, Efim A. Khazanov15, Ryosuke Kodama16, Georg Korn17, Ruxin Li18, Yutong Li19, Jens Limpert20;21;22, Jingui Ma23, Chang Hee Nam24, David Neely8;25, Dimitrios Papadopoulos9, Rory R. Penman1, Liejia Qian23, Jorge J. Rocca26, Andrey A. Shaykin15, Craig W. Siders4, Christopher Spindloe8,Sandor´ Szatmari´ 27, Raoul M. G. M. Trines8, Jianqiang Zhu28, Ping Zhu28, and Jonathan D. Zuegel7 1AWE, Aldermaston, Reading, UK 2OxCHEDS, Clarendon Laboratory, Department of Physics, University of Oxford, Oxford, UK 3CIFS, Blackett Laboratory, Imperial College, London, UK 4NIF & Photon Science Directorate, Lawrence Livermore National Laboratory, Livermore, USA 5Fraunhofer Institute for Laser Technology (ILT), Aachen, Germany 6Chair for Laser Technology LLT, RWTH Aachen University, Aachen, Germany 7University of Rochester, Laboratory for Laser Energetics, Rochester, USA 8Central Laser Facility, STFC Rutherford Appleton Laboratory, Chilton, Didcot, UK 9LULI, CNRS, CEA, Sorbonne Universites,´ Ecole´ Polytechnique, Institut Polytechnique de Paris, Palaiseau, France 10Department of Physics, The Ohio State University, Columbus, USA 11Centre for Ultrafast Optical Science, University of Michigan, Ann Arbor, USA 12Intense Laser Irradiation Laboratory, Istituto Nazionale di Ottica (INO), CNR, Pisa, Italy 13Institute of Optics and Quantum Electronics, Friedrich-Schiller-University Jena and Helmholtz Institute, Jena, Germany 14The Graduate School for the Creation of New Photonics Industries, Nishiku, Hamamatsu, Japan 15Institute of Applied Physics, Russian Academy of Sciences, Nizhny Novgorod, Russia 16Institute of Laser Engineering, Osaka University, Suita, Osaka, Japan 17ELI-Beamlines, Institute of Physics, Czech Academy of Sciences, Prague, Czech Republic 18State Key Laboratory of High Field Laser Physics, Shanghai Institute of Optics and Fine Mechanics, Chinese Academy of Sciences, Shanghai 201800, China 19National Laboratory for Condensed Matter Physics, Institute of Physics, Chinese Academy of Sciences, Beijing 100190, China 20Institute for Applied Physics (IAP) at Friedrich-Schiller-University Jena, Jena, Germany 21Helmholtz Institute Jena, Jena, Germany 22Fraunhofer Institute for Applied Optics and Precision Engineering (IOF), Jena, Germany 23Key Laboratory for Laser Plasma (Ministry of Education), School of Physics and Astronomy, Shanghai Jiao Tong University, Shanghai 200240, China 24Centre for Relativistic Laser Science (CoReLS), Institute for Basic Science, Department of Physics and Photon Science, Gwangju Institute of Science and Technology, Gwangju, South Korea 25SUPA, Department of Physics, University of Strathclyde, Glasgow, UK 26Colorado State University, Fort Collins, Colorado, USA 27Department of Experimental Physics, University of Szeged, Szeged, Hungary 28National Laboratory on High Power Laser and Physics, Shanghai Institute of Optics and Fine Mechanics, Chinese Academy of Sciences, Shanghai 201800, China (Received 10 March 2019; accepted 21 June 2019) Correspondence to: C. N. Danson, AWE, Aldermaston, Reading, UK. Email: [email protected] 1 Downloaded from https://www.cambridge.org/core. 06 Oct 2021 at 13:05:40, subject to the Cambridge Core terms of use. 2 C. N. Danson et al. Abstract In the 2015 review paper ‘Petawatt Class Lasers Worldwide’ a comprehensive overview of the current status of high- power facilities of >200 TW was presented. This was largely based on facility specifications, with some description of their uses, for instance in fundamental ultra-high-intensity interactions, secondary source generation, and inertial confinement fusion (ICF). With the 2018 Nobel Prize in Physics being awarded to Professors Donna Strickland and Gerard Mourou for the development of the technique of chirped pulse amplification (CPA), which made these lasers possible, we celebrate by providing a comprehensive update of the current status of ultra-high-power lasers and demonstrate how the technology has developed. We are now in the era of multi-petawatt facilities coming online, with 100 PW lasers being proposed and even under construction. In addition to this there is a pull towards development of industrial and multi-disciplinary applications, which demands much higher repetition rates, delivering high-average powers with higher efficiencies and the use of alternative wavelengths: mid-IR facilities. So apart from a comprehensive update of the current global status, we want to look at what technologies are to be deployed to get to these new regimes, and some of the critical issues facing their development. Keywords: exawatt lasers; high-power lasers; petawatt lasers; ultra-high intensity 1. Introduction laser in 1960[9]. In the following few years there was vigorous research activity as attempts were made to increase There have been two published reviews of ultra-high-power the peak power and focused intensity in order to reach lasers separated by nearly two decades; the first by Backus extreme conditions within the laboratory. Initial jumps of et al.[1] in 1998, when there was only one petawatt class several orders of magnitude in peak power came with the laser in operation[2], and the second by Danson et al.[3] invention of Q-switching[10], and then mode-locking[11–15]. some 17 years later, which identified approximately fifty Progress slowed, as illustrated in Figure1, until the late petawatt class lasers either operational, under construction 1980s, with the development of the technique of chirped or in the planning phase. These review papers were cited pulse amplification (CPA) by Strickland and Mourou[16] at by the Nobel Committee for Physics in its 2018 award to the Laboratory for Laser Energetics (LLE) at the University Strickland and Mourou in its Scientific Background paper[4] of Rochester, USA. ‘Groundbreaking Inventions in Laser Physics’. In addition A parallel problem existed in radar systems, where short, to these the National Academy of Sciences, Engineering and powerful pulses that were beyond the capabilities of existing Medicine in the USA published a report in December 2017 electrical circuits were needed. Using dispersive delay ‘Opportunities in Intense Ultrafast Lasers: Reaching for the lines, the radar pulses could be stretched and amplified [5] Brightest Light’ , which summarized the current status of prior to transmission, and then the reflected pulse could be high-power lasers, highlighting the decline in this activity compressed, avoiding high-peak powers within the amplifier within the USA in recent years, and making recommenda- circuitry[17]. In the telecommunications industry, work was tions of how this should be remedied. carried out on the use of prisms[18] and grating pairs[19] A special mention should also be made to the work to compensate for the spectral phase distortions imposed of ICUIL. ICUIL, the International Committee on Ultra- on broad bandwidth laser pulses by long lengths of optical High Intensity Lasers, is an organization concerned with fibre. By putting a telescope inside a grating pair, Martinez international aspects of ultra-high-intensity laser science, produced a method to reverse the sign of the spectral phase technology and education. This was formed in 2003 fol- that was imparted, thus creating a device that could stretch a lowing the work of the OECD (Organisation for Economic pulse and then exactly compress it. These systems were used Co-operation and Development) Global Science Forum[6] in stretching pulses prior to propagation along the fibre, then under the International Union of Pure and Applied Physics compressing them in order to reduce nonlinear effects. (IUPAP)[7]. ICUIL has a very active programme of work Strickland and Mourou’s approach was to take the 150 ps and maintains a track of ultra-high-intensity lasers on its output from a commercial mode-locked Nd:YAG oscillator, website[8]. It also hosts a biennial conference to bring the which was then stretched to 300 ps and spectrally broadened community together for the exchange of information on the in 1.4 km of optical fibre, using a combination of group subject. velocity dispersion and self-phase modulation. The pulse was then amplified in a Nd:glass regenerative amplifier, and [20] 1.1. Introduction – historical perspective compressed using a Treacy grating pair which compen- sated for the second-order spectral phase imposed by the The possibility of using lasers to achieve previously un- fibre. From the original CPA paper’s conclusion, it states ‘we obtainable states of matter in the laboratory gained much have shown that by first stretching a chirped optical pulse and attention following the demonstration of the first pulsed then amplifying before compressing, high-peak power pulses Downloaded from https://www.cambridge.org/core. 06 Oct 2021 at 13:05:40, subject to the Cambridge Core terms of use. Petawatt and exawatt class lasers worldwide 3 Figure
Details
-
File Typepdf
-
Upload Time-
-
Content LanguagesEnglish
-
Upload UserAnonymous/Not logged-in
-
File Pages54 Page
-
File Size-