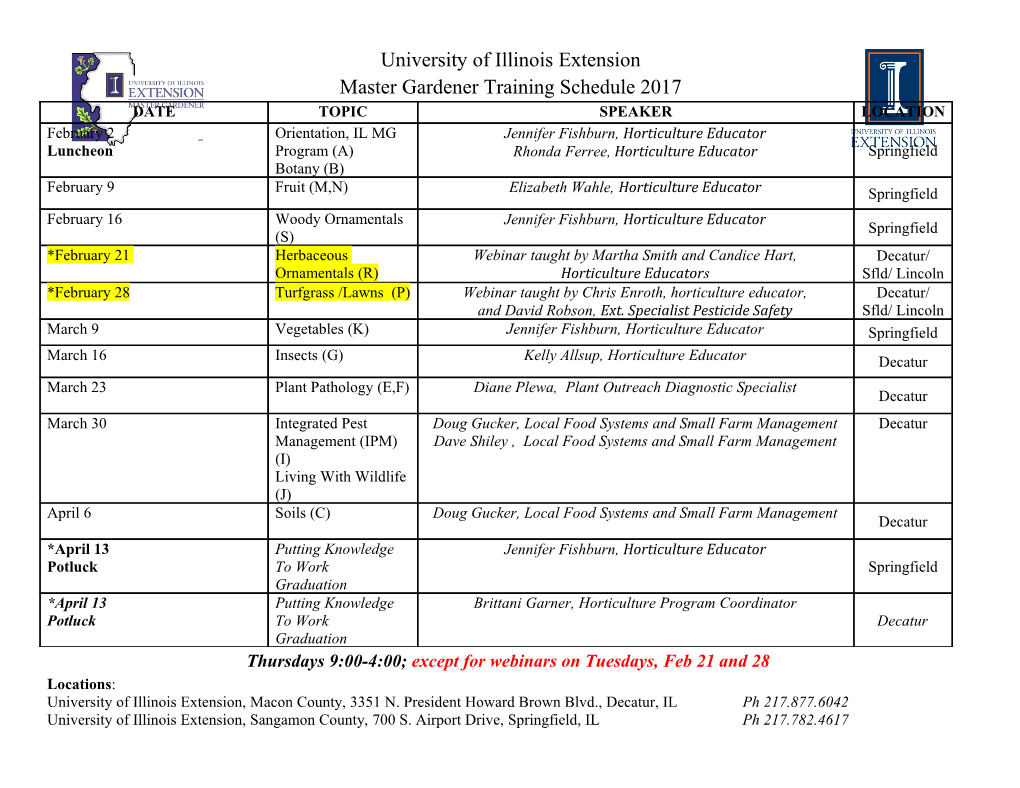
HYDROLOGICAL PROCESSES Hydrol. Process. 17, 1691–1710 (2003) Published online 5 March 2003 in Wiley InterScience (www.interscience.wiley.com). DOI: 10.1002/hyp.1210 Determining long time-scale hyporheic zone flow paths in Antarctic streams Michael N. Gooseff,1* Diane M. McKnight,1 Robert L. Runkel2 and Bruce H. Vaughn1 1 Institute of Arctic and Alpine Research, University of Colorado, Boulder, CO, 80309-0450, USA 2 U. S. Geological Survey, Mail Stop 415, Denver Federal Center, Denver, CO, 80225, USA Abstract: In the McMurdo Dry Valleys of Antarctica, glaciers are the source of meltwater during the austral summer, and the streams and adjacent hyporheic zones constitute the entire physical watershed; there are no hillslope processes in these systems. Hyporheic zones can extend several metres from each side of the stream, and are up to 70 cm deep, corresponding to a lateral cross-section as large as 12 m2, and water resides in the subsurface year around. In this study, we differentiate between the near-stream hyporheic zone, which can be characterized with stream tracer experiments, and the extended hyporheic zone, which has a longer time-scale of exchange. We sampled stream water from Green Creek and from the adjacent saturated alluvium for stable isotopes of D and 18O to assess the significance and extent of stream-water exchange between the streams and extended hyporheic zones over long time-scales (days to weeks). Our results show that water residing in the extended hyporheic zone is much more isotopically enriched (up to 11‰ Dand2Ð2‰ 18O) than stream water. This result suggests a long residence time within the extended hyporheic zone, during which fractionation has occurred owing to summer evaporation and winter sublimation of hyporheic water. We found less enriched water in the extended hyporheic zone later in the flow season, suggesting that stream water may be exchanged into and out of this zone, on the time-scale of weeks to months. The transient storage model OTIS was used to characterize the exchange of stream water with the extended hyporheic zone. Model results yield exchange rates (˛) generally an order magnitude lower (105 s1) than those determined using stream-tracer techniques on the same stream. In light of previous studies in these streams, these results suggest that the hyporheic zones in Antarctic streams have near-stream zones of rapid stream-water exchange, where ‘fast’ biogeochemical reactions may influence water chemistry, and extended hyporheic zones, in which slower biogeochemical reaction rates may affect stream-water chemistry at longer time-scales. Copyright 2003 John Wiley & Sons, Ltd. KEY WORDS isotope transport; OTIS; Dry Valleys; hyporheic zone INTRODUCTION Fluvial hydrological flow paths, and in particular hyporheic exchange processes, have been identified as important for understanding aquatic biogeochemical cycling (Harvey and Bencala, 1993; Mulholland et al., 1997; Gooseff et al., in press). White (1993) noted that there is no single definition or method of delineation of the hyporheic zone that applies for all hyporheic zone studies, although Harvey and Bencala (1993) developed the following hydrological definition: a subsurface flow path parallel to stream flow in which water that was recently in the stream mixes with subsurface water and will shortly return to the stream. As Bencala (2000) points out, ‘hyporheic zones influence the biogeochemistry of stream ecosystems by increasing solute residence times.’ The extent of the actively exchanging hyporheic zone within the adjacent wetted zone of a typical stream cannot be measured directly (i.e. vertical extent versus lateral extent). Current methods for characterizing hyporheic zone influences on stream processes generally require a stream-tracer injection experiment and subsequent inverse modelling to fit model output to observed data using the transient storage * Correspondence to: Michael N. Gooseff, Department of Aquatic Watershed and Earth Resources, Utah State University, 5210 Old Main Hill, Logan, UT 84322-5210, USA. E-mail: [email protected] Received 22 February 2002 Copyright 2003 John Wiley & Sons, Ltd. Accepted 6 August 2002 1692 M. N. GOOSEFF ET AL. (TS) model developed by Bencala and Walters (1983). Harvey et al. (1996) have discussed the reliability of the stream-tracer approach to characterize hyporheic exchange. Their results show that the method is reliable for characterizing ‘near-stream’ flow paths and storage zones, that is, flow paths that exchange on the time- scale of the experiment (generally hours to a few days), and that the technique most reliably characterizes this exchange at low baseflow conditions. The tracer method is not sensitive to slower exchange with storage zones more distant from the stream, because the time-scale of exchange into and out of ‘distant’ hyporheic zones is greater than the duration of the stream-tracer experiment. In this study, we define the hyporheic zone as the area of saturated alluvium adjacent to the stream that exchanges water and solutes, on any time-scale, with the stream. Further, we will distinguish between two hyporheic zones, (i) the near-stream hyporheic zone, which is readily characterized by the stream-tracer technique, as described by Harvey et al. (1996), and has time-scales of exchange on the order of hours, and (ii) the extended hyporheic zone, in which exchange with the stream occurs on the time-scale of days to weeks or longer. We propose that the extended hyporheic zone is tenuously connected to the stream, and we expect that over a long time (weeks to months), the influence of the stream on the extended hyporheic zone, and vice versa is detectable. The streams of the Antarctic Dry Valleys provide a unique environment in which to study fluvial processes. The streams are fed by glacial meltwater during the austral summer, a period of constant sunlight. Because there is no vegetation, the extent of the wetted sediment adjacent to the stream, which comprises the hyporheic zone, can be measured directly, unlike temperate streams. There is no regional groundwater system in contact with the streams (Chinn, 1993), thus all water within the adjacent wetted zone comes from the stream. The exchange of stream water between the stream and the near-stream hyporheic zone has been characterized in two Dry Valley streams in previous tracer experiments: Huey Creek (Runkel et al., 1998) and Green Creek (McKnight et al., 1999). Both studies found that the porous alluvium of the streambeds allowed for rapid rates of near-stream hyporheic exchange. Consequences of rapid exchange between the stream and the near-stream hyporheic zone are increased weathering rates of streambed material (Lyons et al., 1997; Maurice et al., 2002; Gooseff et al., in press) compared with temperate watersheds, and increased nutrient uptake as stream water flows toward the closed-basin lakes on the valley floors (McKnight et al., 1999). Physical cross-sectional wetted areas adjacent to streams are much larger than the storage zone size identified by previous tracer experiments in Dry Valley streams (Conovitz, 2000). We hypothesize that all of this extensive wetted cross- sectional area comprises an actively exchanging hyporheic zone, and that previous tracer methods have not identified long timescale extended hyporheic exchange. Many recent studies have focused on the discretization of water flow paths and water residence times in the environment using stable isotopes (Stout, 1967; Hooper and Shoemaker, 1986; Kennedy et al., 1986; Turner et al., 1987; Maule´ and Stein, 1990; McDonnell et al., 1991; Cooper et al., 1993; Kendall and Caldwell, 1998). Other studies have shown that the flow path water takes and its residence time in the environment (e.g. in the subsurface of a hillslope) strongly influence the quality of water observed in streams (Kennedy, 1971; Pilgrim et al., 1979; Cirmo and McDonnell, 1997). The most commonly used stable isotopes as hydrological tracers are deuterium (D) and 18O. Stable isotopes can be used in hydrograph separation, to discern subsurface flow from overland flow as well as to discern spring snowmelt contribution to stream flow (Cooper et al., 1991, 1993; McDonnell et al., 1991; Stewart and McDonnell, 1991; McNamara et al., 1997). Stable isotopes can also be used to quantify hydrological fluctuations in glacially dominated catchments (Epstein and Sharp, 1959; Theakstone and Knudsen, 1996), and as an indicator of permafrost and active layer contributions to local hydrology (McLean et al., 1999). Modelling isotope transport and fractionation in groundwater systems (Krabbenhoft et al., 1990; Johnson and DePaolo, 1997) has provided a framework for similar studies in other hydrological systems. Studies of stable isotopes with respect to hydrological and atmospheric interactions in temperate streams have been reported. McKenna et al. (1992) investigated bank storage of enriched Lake Tahoe water in the Truckee River, USA. Gremillion and Wanielista (2000) reported evaporative υ18O enrichment by roughly 1‰ of water in the Econlockhatchee River, USA, compared with other watershed sources (groundwater, precipitation). Simpson Copyright 2003 John Wiley & Sons, Ltd. Hydrol. Process. 17, 1691–1710 (2003) LONG TIME-SCALE HYPORHEIC FLOW PATHS 1693 and Herczeg (1991) also studied stream-water enrichment owing to evaporation on the River Murray in southeast Australia. They found that υD enrichment was C0Ð62‰ for 1% loss of water volume. In this study we use stable isotopes of stream and substream water as a natural hydrological tracer to discern the hydrological flow path and the residence times of water in Dry Valley stream systems. We hypothesize that isotopic signatures of the water residing in the extended hyporheic zone will be enriched compared with the isotopic signature of flowing stream water. We further propose that this enrichment in the extended hyporheic zone is probably the result of evaporation (during the summer) and sublimation (during the winter) of the water through the porous sediments. Enrichment of the water from evaporation and sublimation suggests that extended hyporheic water has a longer and slower flow path than water in the stream, allowing ample time for these processes to occur.
Details
-
File Typepdf
-
Upload Time-
-
Content LanguagesEnglish
-
Upload UserAnonymous/Not logged-in
-
File Pages20 Page
-
File Size-