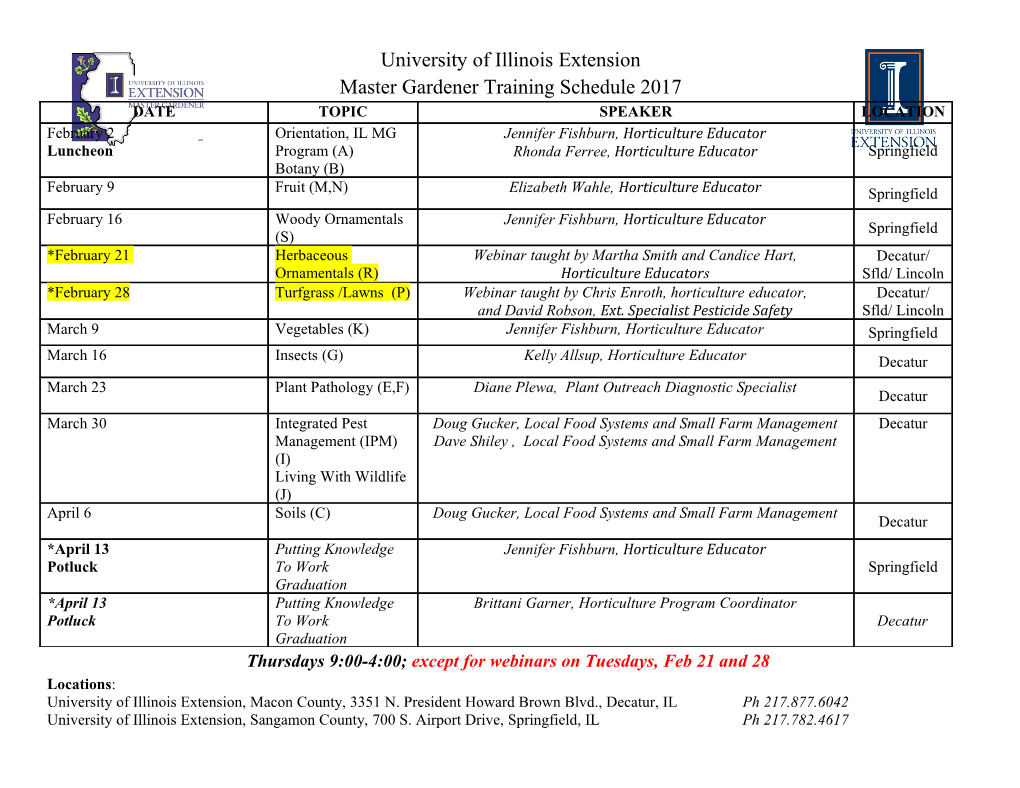
INCOMPATIBILITY AND SPECIATION WITHIN & BETWEEN SPECIES by HANNA MOORE A THESIS Presented to the Department of BIOLOGY and the Robert D. Clark Honors College in partial fulfillment of the requirements for the degree of Bachelor of Science June 2015 Acknowledgements I would like to use this space to thank and acknowledge all those who contributed their skill, knowledge, help, and mental and emotional support to make this thesis a reality. I thank Dr. Patrick Phillips for his mentorship and guidance in designing and carrying out this experiment, as well as his patience and support in helping me to analyze and synthesize my findings. I thank both Dr. Samantha Hopkins and Dr. Peter Wetherwax for their participation on my thesis committee and their general advising and support over the past couple of years. I would like to sincerely thank and acknowledge Valerie Haskins, Chris Hanson, and Addison Kaufman all of whom contributed many volunteered hours of their time in the lab helping me transfer, hatch off, and count the 71,335 worms and 277,850 eggs I analyzed (as well as the extras that weren’t used). I would like to sincerely thank both Miriam Rigby and Christine O’Connor for contributing their computational skills, time, and knowledge and making all of the data figures and edits possible. I thank John Willis and Janna Fierst for working to help me begin the genomics analysis that didn’t quite make it in time to be included. And lastly, but absolutely not least, I thank all of my friends and family who have feigned or expressed real interest in hearing about “my worms” and who have provided their support and encouragement throughout the past three years of data collection and the entire thesis process. iii Table of Contents General Introduction 1 Speciation 1 Reproductive Isolating Mechanisms (RIMs) 2 Population Divergence and the Accumulation of Incompatibilities 5 Implications of the BDM model based on Mendelian Genetics 10 Quantification of Genetic Incompatibility—Phenotypic Indicators 16 Incompatibilities and Geographical Distance 18 Purpose 19 Hypotheses 19 Model Organism 20 Formal Introduction 22 Methodology and Experimental Design 25 Worm Maintenance 25 Phenotypic Data Collection via Crosses 25 Results 28 Male/Female effects 28 Survivorship 32 Fecundity 34 Percent Male Offspring 36 Backcross 38 Discussion 40 Effects due to Parental Background 40 Within- and Between-Species Incompatibilities 42 Survivorship 42 Female Fecundity 44 Percent of Male Offspring 45 Individual Variability 46 Haldane’s Rule and X-effects 47 Conclusions 51 Glossary 53 Bibliography 54 iv General Introduction Earth is home to a staggering amount of diversity. Estimates project that the earth currently houses approximately 8.7 million eukaryotic species (Mora, et al. 2011). If you were to take one second to look at each one of those species (leaving no time to sleep or eat) and keeping in mind that no bacteria are represented, it would take close to 108 days! Yet this projection of the earth’s diversity is not a static figure. In the past few years it has become common to hear discussion of threats to diversity—increased development, pollution, and climate change to name a few—with a focus on factors that cause a decrease in diversity. However, the other side of change in diversity, its increase, is less commonly considered. Not all organisms and species were in place at the moment of the earth’s formation. The first life on Earth is thought to have been single cellular prokaryotic bacteria; yet, 3.7 billion years later this singular event has led to the generation of millions of new species of prokaryotes and eukaryotes (Freeman 2014). Diversification and speciation occurred—new species were generated. Speciation Speciation is the evolutionary process by which a new species is created (Cook 1906). Using the generally accepted, Biological Species Concept understanding of a species, speciation occurs when two populations of organisms are unable to reproduce, and thus are reproductively isolated (Mayr 1942). In studying the incompatibilities between known species, reproductive isolation is found to be caused by the accumulation of genetic and phenotypic incompatibilities (reproductive isolating mechanisms) that gradually lead to the finalizing, and ultimately isolating, step of speciation (Bateson 1909, Dobzhansky 1937, Muller 1958). Is the accumulation of incompatibilities predictable or random? Does distance between populations matter in the rate of accumulation of incompatibilities (Orr 1993)? Are there measurable effects from males or females in terms of reproductive success? These questions are difficult to answer because evolution does not have a clear beginning or end and the processes that underlie speciation can take place over a period of hundreds of years—far longer than any scientist’s lifetime. However, with the right model, these questions can be addressed within an experimental setting in order to derive conclusions that may still apply generally to the natural environment. Answers to these fundamental questions can lead us to a better understanding of the diversification of life on earth, lending new insight into extinct species’ evolution as well as currently diverging populations of species. Reproductive Isolating Mechanisms (RIMs) The incompatibilities that are instrumental in reproductive isolation and speciation can be placed in two general categories—pre-zygotic RIMs and post-zygotic RIMs (Mayr 1963, Seehausen, et al. 2014). A zygote is the initial stage of embryonic development in organisms following the fertilization of the egg by sperm. Thus, RIMs are grouped into two classifications: those that prevent the egg and sperm from meeting, and those that arise following fertilization (Mayr 1963). While pre-zygotic and post-zygotic isolating mechanisms are typically both involved in leading to the final speciation between two groups, generally the process begins with extrinsic, or external environmentally focused factors of post-zygotic 2 isolation (Mayr 1963). In considering the basic life purpose of organisms: to mate, reproduce, and pass on their genes to future generations, pre-zygotic barriers to reproduction are considered to be the more energy efficient of the two methods (Mayr 1963, Futuyma 2013). Pre-zygotic isolation can be caused by a variety of factors. Common pre-zygotic factors range from extrinsic factors such as life history patterns of organisms and environmental differences, to intrinsic structural differences and chemical incompatibilities (Seehausen, et al. 2014). Temporal isolation is caused when organisms are isolated by time of reproduction, or even more generally, time of interaction. Individuals from two tree species may flower and fruit at different times of the year and therefore not have the opportunity to cross-pollinate. Alternatively, a nocturnal animal may never interact with, let alone attempt to reproduce with a diurnal animal (an animal active during the day). Similar to temporal isolation is habitat isolation; like the diurnal and nocturnal animals, organisms that live in different habitats will never have the opportunity to attempt reproduction. Less environment-centered pre-zygotic isolating mechanisms include behavioral and structural isolation. Behavioral isolation is commonly observed in animals with mating rituals such as deer, in male combat, or frogs or birds, in their songs. If an organism is not attracted to a mate by the specific mating behavior, they will not mate. Perhaps most obvious of pre-zygotic RIMs is structural isolation: some organisms are physically unable to mate with each other. While pre-zygotic isolating mechanisms are varied in the way they prevent mating, in terms of energy cost, they all prevent an organism from the costs associated with raising an offspring that may not survive or reproduce. Thus, in the context of the organismal 3 purpose of passing on genes, through pre-zygotic isolating mechanisms, the organism eliminates the chance for wasted energy and is instead able to apply that energy towards successful mating endeavors. Post-zygotic RIMs are more costly as they rely on the expenditure of energy in growing and raising an inviable or infertile offspring. Post-zygotic mechanisms lead to isolation between interbreeding species after the production of inviable hybrid offspring, infertile hybrid offspring, or generally lower fitness hybrid offspring (Mayr 1963, Baird 2002). Inviable offspring include offspring that do not fully develop but may be terminated at some stage in their development, or offspring that are produced with such deleterious genetic or phenotypic mutations that they are unable to function and survive (Mayr 1963). Infertile hybrid offspring do survive but are unable to produce offspring of their own (Mayr 1963, Baird 2002). In both cases any possibility of a future gene line is terminated directly with the hybrid generation. Unlike pre-zygotic RIMs, which are caused by well-established ecological, structural, or behavioral barriers to mating, post-zygotic RIMs are most typically caused by intrinsic post-zygotic isolation (Seehausen, et al. 2014). Although the processes underlying intrinsic post-zygotic isolation are not well understood (as there are a myriad of factors that could cause the inviable or infertile offspring) genetic incompatibility, with its ability to affect and disrupt a host of developmental systems, is believed to be the broader cause (Coyne & Orr 2004). When considering the accumulation of incompatibilities in terms of pre-
Details
-
File Typepdf
-
Upload Time-
-
Content LanguagesEnglish
-
Upload UserAnonymous/Not logged-in
-
File Pages60 Page
-
File Size-