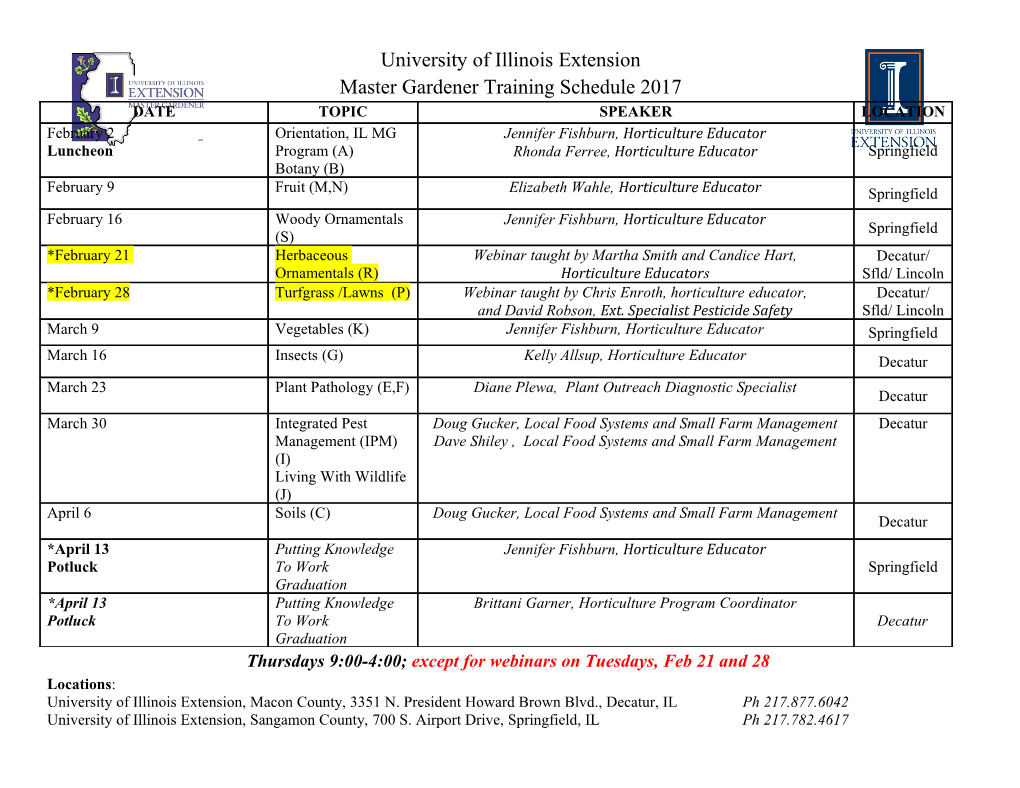
Chapter 26: Fluid, Electrolyte, and Acid-Base Balance Chapter 26 is unusual because it doesn’t introduce much new material, but it reviews and integrates information from earlier chapters to cover 3 types of regulation: regulation of fluid volume, regulation of electrolyte (=ion) concentrations, and regulation of pH. • Outline of slides: • 1. Regulating fluid levels (blood/ECF) • Compartments of the body • Regulation of fluid intake and excretion • 2. Regulating ion concentrations (blood/ECF) • 3. Regulating pH (blood/ECF) • Chemical buffers • Physiological regulation • Respiratory • Renal 1 3 subsections to this chapter – we will cover the middle one only briefly. 1 Ch. 26: Test Question Templates • Q1. Given relevant plasma data, classify a patient’s possible acid-base disorder as a metabolic or respiratory acidosis or alkalosis that is or is not fully compensated. Or, if given such a disorder, give expected plasma pH and CO2 level (high, normal, or low). • Example A: Plasma pH is 7.32, CO2 levels in blood are low. What is this? • Example B: A patient’s plasma has a pH of 7.5. Explain how you could make an additional measurement to determine whether the cause of this unusual pH is metabolic or respiratory. • Example C: A patient’s plasma CO2 levels are very low, yet plasma pH is normal. How can this be? 2 Q1. Example A: (slight) metabolic acidosis. Example B: Measure the CO2 level in the plasma. If the high plasma pH is due to a respiratory problem, the CO2 concentration will be low. If the high pH is NOT due to a respiratory problem, the CO2 will not be low, and may be high if the person is undergoing respiratory compensation for a metabolic alkalosis. Example C: low CO2 levels should normally cause pH to be high, but the normal + pH suggests that either the low CO2 is a compensation for low H secretion and low - + - HCO3 reabsorption, or vice versa (i.e., the low H secretion and low HCO3 reabsorption are a compensation for low CO2). *** Consider an even broader treatment next time? Include cases where CO2 levels are normal but pH is not? 2 • Q2. Given a specific perturbation to a patient’s extracellular fluid, predict the patient’s response in terms of autonomic nervous system output and hormone secretions. • Example. A patient’s body fluids were normal; then she drank a huge amount of pure water. How will this affect the patient’s secretion of antidiuretic hormone and angiotensin II? Explain your reasoning. • Q3. Given a diagram of RAAS, answer questions about it. • Example. Look at Marieb & Hoehn Figure 19.12. What enzymes, if any, are needed to create angiotensin-II? 3 Q2. A large influx of pure water will (at least temporarily) lower ECF fluid osmolarity and raise ECF volume and blood pressure. Since angiotensinogen gets converted to angiotensin II when blood pressure is low, angiotensin II will NOT be elevated in this case. Similarly, since ADH is released in response to high plasma osmolarity or low blood pressure, it will not be elevated either. Q3. Two enzymes are shown here: renin (to convert angiotensinogen to angiotensin-I) and ACE (to convert angiotensin-I to angiotensin-II). 3 Part 1: Regulation of body fluid [an example to illustrate the challenge of body fluid homeostasis] • At rest, the human heart’s cardiac output is ~5000 mL/min (5 L/min). • ~20% of all cardiac output goes to the kidneys, so the kidneys receive 1000 mL/min. • ~50% of all blood is plasma, and ~20% of plasma is filtered from the glomeruli into nephron tubules. • Thus, if there was no reabsorption of water, you would produce 1000*0.5*0.2 = 100 mL of urine PER MINUTE (144 L/day). • Q1. How much urine do you actually produce per day? • Q2. Given Q1, how good is your body at fluid regulation? 4 Q1. Most people produce 1-2 liters per day. Q2. VERY good! 4 Fluid is spread among 3 compartments Amerman (2016), Figure 25.2 (like Marieb & Hoehn Figure 26.1) 5 We note on the next slide that plasma + interstitial fluid = ECF. 5 What is the chemical composition of the fluid in these compartments? Q1. How similar, chemically, are the plasma, interstitial fluid, and intracellular fluid? Q2. Does it matter whether chemoreceptors sense plasma or interstitial fluid? Q3. Given Q1-Q2, do we have a term for plasma + ISF? Marieb & Hoehn (2019), Figure 26.2 6 Q1. The plasma and interstitial fluid are quite similar to each other (except for protein anions) and quite different from the intracellular fluid. Q2. Chemoreceptors would report similar results in either location. (Baroreceptors would report very different results, though. To monitor blood pressure you’d need to have the receptors in the blood itself.) Q3. Extracellular fluid (ECF) is plasma + interstitial fluid. 6 How do we sense and control ECF volume? • We can’t directly sense it. (We don’t have an internal graduated cylinder…) • Two variables provide indirect indications. • Blood pressure • If BP is low, ECF volume may be low too. • ECF osmolarity • If osmolarity is high (e.g., due to sweating), volume may be low too. • If we keep BP and ECF osmolarity near their setpoints, ECF volume should be OK too. Regulated variable Receptor type Receptor location(s) Blood pressure ECF osmolarity 7 Read through this slide. *** BP: baroreceptors; aortic arch, carotid artery, afferent arterioles (JG cells) in kidneys. Osmolarity: osmoreceptors; hypothalamus. 7 Details: osmoreceptors (in hypothalamus) Q1. Which receptors sense changes in cell size/shape? (A) chemoreceptors, (B) mechanoreceptors, (C) nociceptors, (D) photoreceptors, (E) thermoreceptors Q2. Are osmoreceptors neurons? Image: Physiology of Behavior by Neil R. Carlson 8 How can the body sense osmolarity, the sum of all solutes? Follow the water. *** Q1. mechanoreceptors. Q2. Yes. “Change in firing rate of axon” is referring to action potentials, which only neurons do. (Also, sensory receptors are always neurons except for most of the special senses.) 8 Now: How do the receptors lead to effector responses? Q1. What conditions would cause the most thirst? Marieb & Hoehn (2019), Figures 26.5 and 26.6 9 Increased ECF osmolarity => osmoreceptors => thirst and ADH. *** Low BP => baroreceptors => ADH … and angiotensin-II?! *** Q1. High ECF osmolarity combined with low BP would cause maximum activation of the hypothalamic thirst center. *** So what’s the deal with angiotensin-II? Is it just another way of increasing thirst? No, there’s more… 9 Integrated control of blood pressure and ECF osmolarity: the Renin-Angiotensin-Aldosterone System (RAAS) GFR = glomerular filtration rate. Blood vessel Amerman (2016), Figure 24.14 (like Marieb & Hoehn Figure 19.12) 10 Start with the big picture: input is low BP and output is angiotension-II, which corrects the low BP (see next slide). Then do the details. *** 2 enzymes here! (Note convention that names hover above the reactions they catalyze.) *** JG cells (also called granular cells) are in the afferent arteriole wall (macula densa cells are in the tubule wall). The JG cells are the ones that directly sense BP and release renin if BP is low. 10 What does Angiotensin-II do? Redundant and non-redundant effects Marieb & Hoehn (2019), Figure 19.12 11 Osmolarity changes also promote ADH release and thirst, but there are two additional effects shown here too: aldosterone release and vasoconstriction. *** Additional effect of AT-II not shown in figure: increased sodium reabsorption in the PCT. 11 2 types of fluid loss: how does bleeding compare to sweating? BLEEDING SWEATING Solute concentration of fluid lost (hypotonic, isotonic, or hypertonic to blood) Change in blood osmolarity? Change in blood pressure? Will RAAS be activated? Will person be thirsty? Will ADH be released? 12 Fluid lost: isotonic (B), hypotonic (S). Blood osmolarity: no change (B), increases (S). BP: drops (B), drops (S). RAAS activation: yes (B), yes (S). Thirsty: somewhat (B), YES! (S). ADH: somewhat (B), YES! (S). 12 Further perturbations to blood volume and ECF osmolarity… What would happen if a patient who had lost blood was given pure water, rather than isotonic saline? Q1. Immediate effect on blood pressure? Q2. Immediate effect on osmolarity? Q3. Response to altered osmolarity? 13 Q1. BP rises. Q2. Osmolarity drops. Q3. Osmoreceptors sense lower osmolarity => person not thirsty. ADH not secreted => copious dilute urine. The extra H2O essentially gets peed out. 13 Part 2: Regulating ion (electrolyte) concentrations This is important … but consequences are not always straightforward! Marieb & Hoehn (2019) 14 Part 2 (next 3 slides): regulating ion levels. 14 Q1. Find the hormones listed in the table that are important in regulating Na+, K+, and Ca2+ levels. Q2. As we have seen, calcium plays important roles in motor neurons, skeletal muscle, and cardiac muscle. Before consulting the table, predict the consequences of hypercalcemia. Q3. Now look at the table. Was your prediction right? (It’s OK if not!) Marieb & Hoehn (2019) 15 Q1. Na+ and K+: aldosterone. Ca2+: PTH and vitamin D. Q2. Answers will vary, but one might guess that hypercalcemia could lead to excessive muscle contraction, excessive neurotransmitter release, etc. Q3. Answers will vary, but, perhaps in contrast to some expectations, hypercalcemia results in DECREASED activity of motor neurons and muscle cells. (This is because calcium can block sodium channels, interfering with depolarization.) *** Bottom line: if these ions get too concentrated or too dilute, there are symptoms (not always easy to link to the ion perturbation per se). 15 Summary of Na+ and K+ regulation (one simple example of electrolyte regulation) Q1. Where along the nephron do aldosterone’s effects occur? Q2. Would Addison’s Disease cause hypernatremia, hyperkalemia, both, or neither? Marieb & Hoehn (2019), Figure 26.8 16 Q1.
Details
-
File Typepdf
-
Upload Time-
-
Content LanguagesEnglish
-
Upload UserAnonymous/Not logged-in
-
File Pages23 Page
-
File Size-