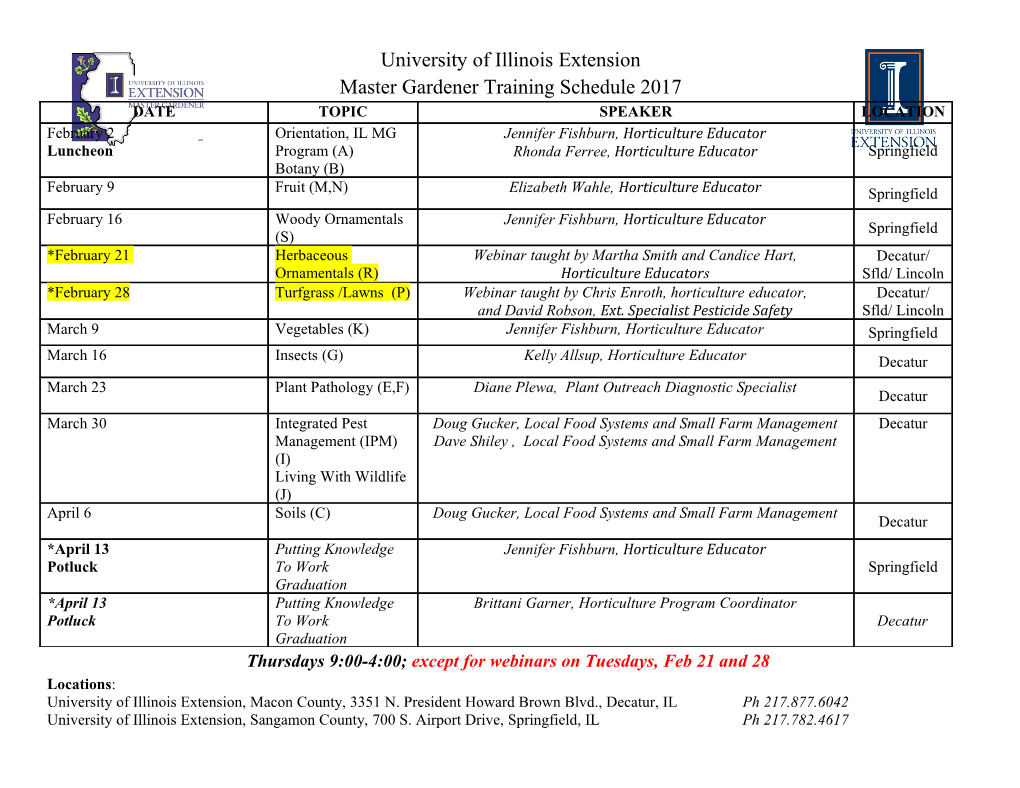
Star Formation and the Origins of Planetary Systems Lecture 10: Icy bodies in outer solar system & debris disk evolution Background reading: Credit: NASA Luu & Jewitt 2002, ARA&A ; Wyatt 2008, ARA&A; Hughes et al. 2018, ARA&A Thanks to M. Wyatt for slides on debris disks Logistical roundup (1/2): Problems accessing previously emailed link to lecture recordings were caused by default browser cookie settings. Probably possible to fix that, but easier solution below... Recorded Kaltura Live Room lectures are here now! Logistical roundup (2/2): Remaining questions from SPF #9 will be answered at the end of this lecture, not during the lecture summary. Please sign up for a time slot to give your presentation (via Doodle poll to be sent by Ewine) . Star Formation and the Origins of Planetary Systems Lecture 10: Icy bodies in outer solar system & debris disk evolution Background reading: Credit: NASA Luu & Jewitt 2002, ARA&A ; Wyatt 2008, ARA&A; Hughes et al. 2018, ARA&A Thanks to M. Wyatt for slides on debris disks 486958 Arrokoth • Classical Kuiper Belt Object • farthest & most primitive object visited by spacecraft • 21 + 15 km contact binary Lecture 10: Icy bodies in outer solar system & debris disk evolution Do you want to build a snowman? Background reading: Credit: NASA, Arrokoth Luu & Jewitt 2002, ARA&A ; Wyatt 2008, ARA&A; Hughes et al. 2018, ARA&A Thanks to M. Wyatt for slides on debris disks Quiz: What does the size of the contact binary components tell you about their collision velocities? Summary Lecture 9 Key point(s) Key figure/table 2. Planetesimal to Model planetesimal swarm statistically with distributions of important parameters. For a select few objects, achieve runaway growth from planetesimals into embryos up to isolation mass, embryo growth defined by Hill sphere. 3a. Terrestrial Isolation mass embryos in inner disk up to ~0.1 Mearth (from #2). Then need gravitational planet formation interactions to push embyos onto colliding orbits. Giant impact phase responsible for many scenario "odd features" of Solar System's terrestrial planets. Earth took ~80 Myr to form. In contrast, Jupiter took <3.8 Myr to form. Two classical models for gas giant formation: core 3b. Gas giant planet accretion & gravitational instability. Former takes nearly too long, latter requires massive, cold formation models disk at correct time in disk evolution. Alternative model: pebble accretion! Combined with streaming instability, it can solve most timescale problems for formation. Three kinds of migration within gas disk, depending on mass ratio of planet to star and disk to star. Type I moves planets inward; II & III are not exclusively in one direction. Observables of 4. Planet migration migration are spiral arms driven by planets and gaps (for certain sizes). Migration of planet can excite high eccentricities/inclinations in smaller planetesimals, leading to impacts. !7 SPF course progress: where are we now? Date SPF # Who Lecture 18 Feb. 1 EvD Motivation, history, & observational facilities 20 Feb. 2 EvD Pre-stellar cores, cloud collapse 25 Feb. 3 EvD SEDs, embedded protostars 27 Feb. 4 EvD Outflows & jets 3 Mar. 5 EvD High mass star formation 5 Mar. P1 TAs Practice exercises #1 (Star formation) 10 Mar. 6 MM Pre-main sequence star types, stellar birthline 12 Mar. 7 MM Circumstellar disks 24 Mar. 8 MM Disk evolution & planet formation (Planet formation) 26 Mar. 9 MM Planet formation: Jovian vs terrestrial planets 31 Mar. 10 MM Icy bodies in outer solar system, debris disk evolution 2 Apr. 11 EvD Condensation processes, meteorites, missions 7 Apr. 12 EvD Chondrules, planetary atmospheres 9 Apr. 13 MM Extrasolar planets 14 Apr. P2 TAs Practice exercises #2 16 Apr. 14 MM Astrobiology (Life formation) !8 Disk evolutionary stages overview SPF #8 Stage 1) Envelope infall 2) Viscous evolution 3) Disk clearing • Matter falls onto disk faster • Infall rate lower. Disk evolves viscously, Disk material already accreted than can be redistributed. under the action of its own friction. by protostar and protoplanets, Features • Global instabilities possible. • Outward angular momentum transport or driven away by energetic processes: • Disk grows with time. allows matter to fall in to star. (winds, photoevap., tidal encounters) 5 6 7 Depends on disk mass & environment: tff : ~ Few x 10 yr ~Few x 10 yr, up to 10 yr Timescale 103 − 107 yr after stage #2. SED Class Class 0, early Class I Late Class I, Class II (opt. thick disk) Class III (opt. thin disk) Pre-main sequence stars: Pre-main sequence star: Protostar Weak-lined T Tauris Star type Classical T Tauri − Herbig AeBe (Towards zero-age main sequence) Lectures SPF #3, #6 SPF #3, #6, #7, #8, #9 SPF #10 !9 Disk evolutionary stages overview SPF #8 Stage 1) Envelope infall 2) Viscous evolution 3) Disk clearing • Matter falls onto disk faster • Infall rate lower. Disk evolves viscously, Disk material already accreted than can be redistributed. under the action of its own friction. by protostar and protoplanets, Features • Global instabilities possible. • Outward angular momentum transport or driven away by energetic processes: • Disk grows with time. allows matter to fall in to star. (winds, photoevap., tidal encounters) 5 6 7 Depends on disk mass & environment: tff : ~ Few x 10 yr ~Few x 10 yr, up to 10 yr Timescale 103 − 107 yr after stage #2. SED Class Class 0, early Class I Late Class I, Class II (opt. thick disk) Class III (opt. thin disk) Pre-main sequence stars: Pre-main sequence star: Protostar Weak-lined T Tauris Star type Classical T Tauri − Herbig AeBe (Towards zero-age main sequence) Lectures SPF #3, #6 SPF #3, #6, #7, #8, #9 SPF #10 !10 2) Defining debris disks Haisch et al. (2001) (SPF#3, 8) Hernandez et al. (2007) (SPF#7) 50% 1) Disk clearing stage 2 Myr "protoplanetary" disk "debris" disk !11 (gassy) (rocky) 3) Solar System's Kuiper Belt & Oort Cloud 1. Protoplanetary disk evolutionary stage #3: disk clearing 2. Debris disks & their evolution 3. Analyze Solar System's Kuiper Belt & Oort Cloud as preserved remnants of #1 & #2 Disk clearing stage timescales Haisch et al. (2001) ▪ Jovian planet formation and migration must occur Hernandez et al. (2007) while gas still present in disk. ▪ Near-IR warm dust surveys show decreased fraction (Near-IR) of stars with disks in clusters after ~2 Myr SPF#7 Ansdell et al. (2016, 2017) 2 Myr ~1.5 Myr (Sub-mm) ≤3 Myr ▪ Some decrease also found in longer wavelength ≤5 mid- and far-IR surveys, but mm surveys show ≤10 Myr decrease only after 3 Myr, with some (cold outer) disks surviving up to 10 Myr. Disk evolution and dispersal, as seen through SEDs Multiple paths from protoplanetary (gas-rich, ‘thick’) to debris (gas-poor, ‘thin’) disks: Grain growth Disk + dispersal? SPF#8 Class II Class II Star ? Gap formation? SPF#8, 9 Class II Class III Merin et al. Quiz: In what order does the disk clear out in the sequence below? inner middle outer Hartmann et al. (2016) Disk Class II Star Class II Class III Mechanisms for disk dispersal ▪ Accretion onto star ▪ Material used for planet formation ▪ Stellar winds ▪ Photoevaporation ▪ External (e.g., Orion) ▪ stellar UV ▪ Close encounters with other stars ⇒ stripping Merin et al. Hollenbach et al. 2000, PPIV Photoevaporation Stellar or external UV heats surface layer of disk to high temperatures ⇒ thermal sound speed large enough to overcome gravitational pull ⇒ flow If layer stays neutral (PDR) ⇒ gas ≈ several 103 K If layer ionized ⇒ gas ≈ 104 K (SPF#6) photo- accretion evaporation Hollenbach et al. 2000, PPIV UV switch Alexander et al. (2014) Once accretion rate drops and cannot keep up anymore with photoevaporation rate, inner disk drains quickly "transition disk" = disk with inner hole or gap. Sometimes see "pre-transition" disk as gapped vs with hole. Hollenbach et al. (2000) 1. Protoplanetary disk evolutionary stage #3: disk clearing 2. Debris disks & their evolution 3. Analyze Solar System's Kuiper Belt & Oort Cloud as preserved remnants of #1 & #2 Debris disks Debris disks are (1) older disks which have (2) lost their gas and where dust is produced in situ by (3) collisions of planetesimals. Merin et al. Debris disks were originally the ‘Vega phenomenon’ ▪ Main sequence (MS) star, Vega (= α Lyrae) with circumstellar dust grains ▪ Calibration star for IRAS satellite → expected to see weak emission. Instead a powerful IR source was found, orders of magnitude brighter at 60 and 100 µm than Rayleigh- the photospheric emission Jeans tail Vega ▪ Vega was thus the first MS star apart from the Sun to have surrounding solid material. ▪ Thermal balance: small grains become hotter than big grains Aumann et al. (1984), Backman & Paresce in PPIII The first debris disk detections with IRAS satellite (1984): the Fab Four. Rayleigh- ▪ Spatially resolved Jeans tail Vega even by IRAS 60 µm Fomalhaut ▪ Distance well known ▪ No near-IR excess β Pic ε Eri (Aumann et al. 1984) Courtesy: Paul Kalas, www.disksite.com Grain sizes and mass ▪ Although planetesimals have formed in these disks, some fraction of grains must be smaller than ~100 µm to emit far-IR radiation ▪ Values of grain size and total grain area depend on emissivity exponent β or grain size distribution exponent m -3 -3 -2 ▪ Minimum mass: all grains have size d=d0 with density 1 gr cm → small masses 10 – 10 MEarth ▪ Maximum mass: assume grain size distribution n(d)∝d-3.5 produced by collisional fragmentation, -3 with dmin=d0, dmax=1000 km (size of gravitational instability at 100 AU) and density 5 gr cm → large masses up to 0.1 MSun Grain removal ▪ Small grains with 0.1 < d < 1 µm are ejected quickly (~104 yr) from system by radiation pressure.
Details
-
File Typepdf
-
Upload Time-
-
Content LanguagesEnglish
-
Upload UserAnonymous/Not logged-in
-
File Pages90 Page
-
File Size-