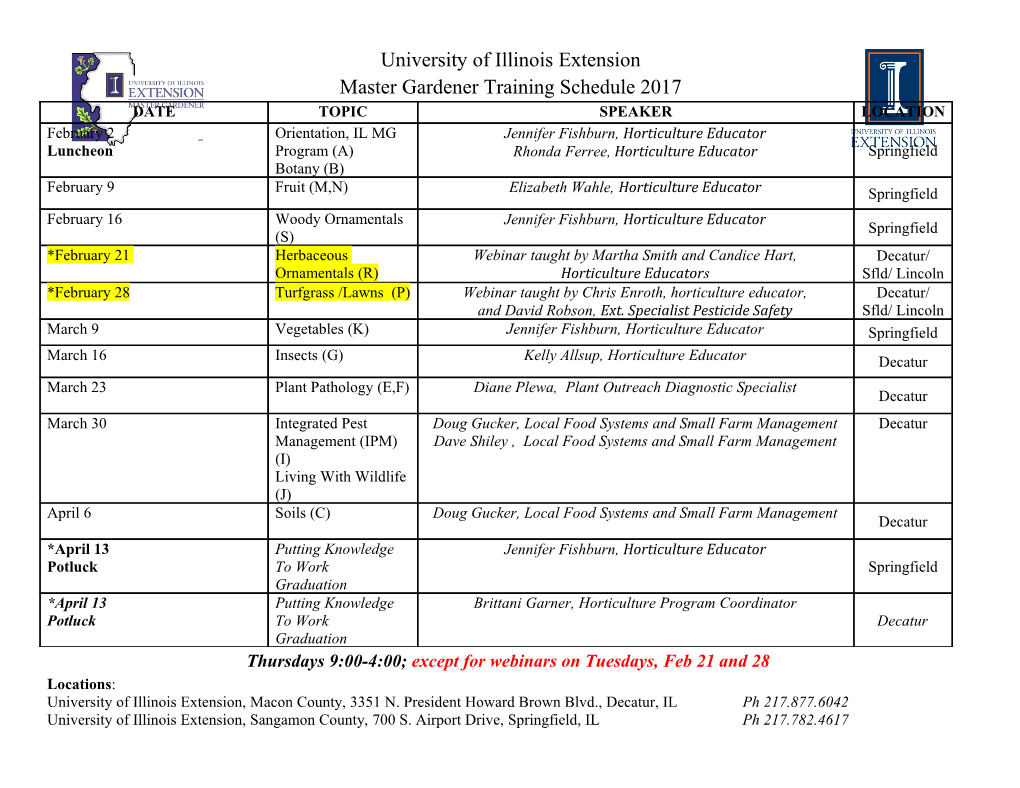
Ester Dance Reaction on the Aromatic Ring Kaoru Matsushita, Ryosuke Takise, Kei Muto, and Junichiro Yamaguchi* Department of Applied Chemistry, Waseda University, 3-4-1, Ohkubo, Shinjuku, Tokyo, 169-8555, Japan ABSTRACT: Aromatic rearrangement reactions are useful tools in the organic chemist’s toolbox when generating uncommon substitution patterns. However, it is difficult to precisely translocate a functional group in (hetero)arene systems, with the exception of halogen atoms in a halogen dance reaction. Herein, we describe an unprecedented “ester dance” reaction: a predictable translocation of an ester group from one carbon atom to another on an aromatic ring. Specifically, a phenyl carboxylate substituent can be shifted from one carbon to an adjacent carbon on a (hetero)aromatic ring under palladium catalysis to often give a thermodynamically favored, regioisomeric product with modest to good conversions. The obtained ester moiety can be further converted to various aromatic derivatives through the use of classic as well as state-of- the-art transformations including an amidation, acylations and decarbonylative couplings. Dedicated to Professor Kuniaki Tatsuta on his 80th birthday. Substitution reactions on (hetero)aromatic substrates are some of there are currently no reports of a catalytic carbonyl group rear- the most important reactions in organic chemistry. However, some rangement. Much like the halogen dance reaction wherein a func- substitution patterns can be more difficult to forge than others based tional group is translocated from one ring carbon to another, we de- on the propensity of the positions on the (hetero)arene to engage in scribe a palladium-catalyzed translocation of an ester group on the electrophilic, nucleophilic or radical-based substitution. In such aromatic ring, coined herein as an “ester dance” reaction. cases, aromatic rearrangement reactions can help access ring posi- During our recent efforts in the development of decarbonylative tions that are difficult to functionalize otherwise. Although there are transformations of aromatic esters (9–13), we found that a phenyl many well-known aromatic rearrangements such as the Smiles, carboxylate at the C1-position of phenyl 1-naphthoate (1a) mi- Claisen, and Bamberger rearrangements, these transformations grated to the C2-position, affording phenyl 2-naphthoate (2a) un- leave behind a functional group at the carbon atom that was origi- der palladium catalysis, albeit with low conversion (18% yield of 2a, nally substituted in the starting material (Fig 1A). A more rare type Fig. D). We hypothesize that product 2a can potentially arise from a of aromatic rearrangement involves translocation of a functional formal 1,2-rearrangement of the ester (phenyl carboxylate) in 1a, in- group, in which the carbon atom bearing the functional group in the volving: (1) oxidative addition of palladium into the ester C(O)–O starting material loses the group entirely, and the same group is bond; (2) deprotonation (ortho C–H bond activation of complex brought back to another carbon atom on the aromatic ring. One ex- A) followed by decarbonylation (14), producing an aryne-palladium ample of this type of aromatic translocation is transalkylation, which complex (or a h2-arene–palladium complex; complex B) (15–18); is a disproportionation of an alkyl group under Friedel–Crafts alkyl- (3) protonation and carbonylation; and (4) reductive elimination. ation conditions, also known as a carbon-substituent rearrangement The product 2a is thermodynamically more stable than reactant 1a (Fig. 1B) (2–4). This reaction can translocate an alkyl substituent to (3.7 kcal/mol lower in energy, Figs. S6 and S7), and it is likely that another position on the same aromatic ring or onto another aromatic this is a reversible reaction that thermally converges to 2a. The prod- ring, allowing for several compounds including isomers to form from uct in this experiment is an ester that can be reacted with nucleo- a single starting material; therefore, it is utilized mainly in the petro- philes using versatile reactions such as metal-catalyzed decarbonyla- chemical industry. However, this reaction requires extremely harsh tive coupling reactions (10–13,19–24), amidation (25) and ketone conditions and unpredictably generates a mixture of products. formation (26,27) (see Fig. S1, more details). Therefore, we began An intriguing example utilized in the synthesis of fine chemicals is to examine to develop this unprecedented reaction. called the “halogen dance” reaction of haloarenes (Fig. 1C) (5–7). After extensive investigation, we found the optimal conditions: This reaction is a translocation induced by a strong base, and pro- when the reaction was conducted with 10 mol% PdCl2, 20 mol% vides a regioisomeric product via a sequence of halogen-metal ex- dcypt [3,4-bis(dicyclohexylphosphino)thiophene](28), and K2CO3 change processes on the aromatic ring. The net effect is a removal of (0.5 equiv) in m-xylene at 150 ºC for 24 h, 1a was converted to 2a in a halogen atom from one ring carbon, and placement onto another 85% yield along with recovered 1a (7% yield) (Table 1, standard ring carbon. Due to its practical and predictable nature, this reaction conditions; also, see Tables S1–S9).Without PdCl2, dcypt, or K2CO3, is often exploited even in the total synthesis of complex natural prod- the reaction completely shut down (Entries 1–3). Other palladium ucts (7). In an example of a simultaneous, multiple rearrangement salts also worked, albeit with a lower yield of 2a; other metal salts on an aromatic ring, Scott and coworkers observed a rearrangement such as NiCl2 were ineffective (Entries 4 and 5). Other electron-rich of boron substituents in an iridium-catalyzed borylation with cata- bidentate ligands such as dcype and dcypbz were also effective, but lytic potassium tert-butoxide (8). To the best of our knowledge, the yields were slightly lower (Entries 6 and 7). Further A. Aromatic rearragement and translocation Y X X Y X X Table 1. Selected optimizations of reaction conditions. Rearrangement Y or O OPh 10 mol% PdCl O OPh Ar Ar’ Ar’ and/or Ar’ 2 O 20 mol% dcypt many reports 1 1 2 K CO (0.5 equiv) Y 2 3 + OPh e.g. Smiles e.g. Claisen e.g. Bamberger m-xylene (0.5 M) X 150 °C, 24 h 1a 1a: 7% recovered 2a: 85% yield Translocation X Standard conditionsa Ar Ar or Ar or Ar few reports X X GC yield (%)b deviation from S entrya the standard conditions 1a 2a B. Transalkylation OH 1 without PdCl2 74 0 P P Me OH Me 2 without dcypt 73 0 Me H+ dcypt cat. Me 3 without K2CO3 86 0 + Me 4 PdBr2 15 51 5 NiCl2 55 1 6 dcype 14 72 Me Me Me Me 7 dcypbz 23 67 8 dppe 78 0 9 dppf 78 0 P P C. Halogen dance reaction Br Li 10 BINAP 79 0 dcype 11 PCy3 86 0 Br Br Br Br H 12 ICy 67 0 H Li Ar Ar 13 Xphos 66 0 LDA + SM H O Br c 2 14 Na2CO3 79 6 Ar Ar Ar c 15 Cs2CO3 0 0 Li Br c 16 K3PO4 65 16 SM H H 17 toluene 58 28 P P Ar Ar 18 1,4-dioxane 42 44 dcypbz 19 DMF 6 5 D. Ester dance reaction (This work) a 1a (0.4 mmol), PdCl2 (10 mol%), dcypt (20 mol%), K2CO3 (0.2 b O OPh O OPh mmol), m-xylene (0.8 mL), 150 ºC, 24 h GC yield was determined by O c 1 cat. Pd 1 2 using n-decane as an internal standard. Base (1.5 equiv) was added. base + OPh 150 °C, 24 h 1a 1a: 60% recovered 2a: 18% yield Generally, this reaction formed the corresponding product 2 (42– 71% yields) along with small amounts of recovered starting material (5–20% yields), as well as hydrolyzed benzoic acid derivatives (5– Complex A Complex B 10% yields). Starting materials with benzo[d][1,3]dioxol-5-yl (1k), Oxidative Deprotonation Protonation addition Decarbonylation Carbonylation; naphthyl (1l) and pyridyl groups (1m) were tolerated under these Reductive elimination reaction conditions, producing the corresponding regioisomers 2k– Complex A Complex B L 2m. Pd L L O OPh Next, we examined this translocation reaction on heteroarene Pd Pd H CO CO cores under the optimized conditions. To this end, isonicotinates or HOPh HOPh (C4-carboxylated pyridines) were found to be applicable in this re- ortho C–H bond activation aryne-Pd η2-arene Pd action, with the ester substituents migrating from the C4- to the C3- position of the pyridine, giving nicotinate products. The use of phe- Fig. 1. (A) Aromatic rearrangement and translocation. (B) Transal- nyl (1n), p-methoxyphenyl (1o) and p-fluorophenyl (1p) isonico- kylation of aromatics. (C) Halogen dance reaction of (hetero)aromatics. tinates afforded the corresponding nicotinates 2n–2p with moder- (D) Discovery of an “ester dance” reaction of aromatic esters, and a ate conversions. When an electron-donating group was present, the plausible mechanism. direction of the isomerization interestingly changed: 2-methoxy- isonicotinate (2q) and 2-ethoxyisonicotinate (2r) were obtained in 61% and 47% isolated yields from the corresponding nicotinates via nearly shut down completely when the base was changed from this translocation reaction. p-Anisyl 2-methoxynicotinate (1s) also K2CO3 (Entries 14–16); assisted deprotonation during the ortho C– gave the C4-isomer 2s in good yield. With benzo-fused heteroarenes, H bond activation of complex A is likely to be important. When us- phenyl quinoline-4-carboxylate (1t) gave C3-isomer 2t in good ing other solvents, toluene or 1,4-dioxane showed successful reac- yield, and phenyl benzo[b]thiophene-2-carboxylate (1u) was also tions but the yields were diminished (Entries 17–19). converted into C3-isomer 2u, albeit with reduced yield (27%).
Details
-
File Typepdf
-
Upload Time-
-
Content LanguagesEnglish
-
Upload UserAnonymous/Not logged-in
-
File Pages5 Page
-
File Size-