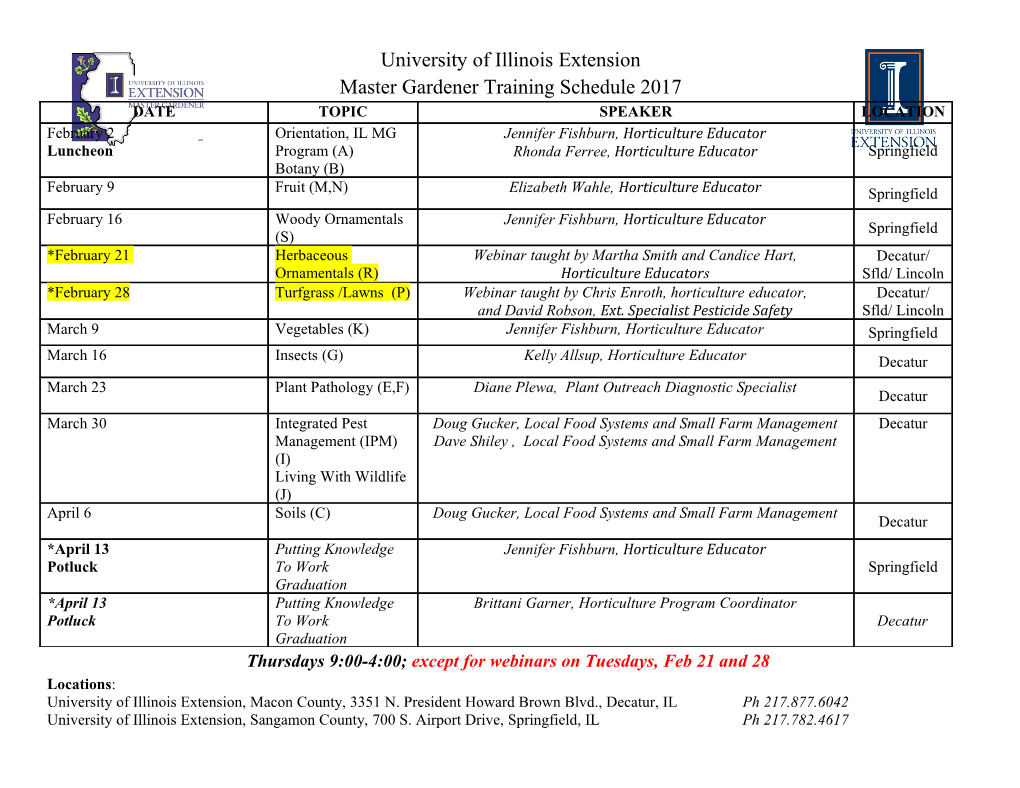
Mars before the space age BARRIE W. JONES Astronomy Group, The Open University, Milton Keynes, MK7 6AA, UK Email: [email protected] Phone: +44 1908 653229 Fax: +44 1908 654192 (SHORT TITLE: Mars pre space age) THIS ARTICLE HAS BEEN PUBLISHED IN THE INTERNATIONAL JOURNAL OF ASTROBIOLOGY, VOLUME 7, NUMBER 2, 143-155 (2008) IJA Mars pre space age + Figs BWJ Version 05Nov2008 1 of 28 13/11/2008 19:30 UT Abstract Mars has surely been scrutinised since the dawn of humankind. Its appearance every couple of years like a drop of blood in the sky, led to warlike attributes in the ancient world. In the 16th century Tycho Brahe made accurate observations of the position of Mars, that enabled Johannes Kepler to obtain his first two laws of planetary motion. These in turn were explained by Newton’s laws of motion and gravity. In the 17th century the first telescope observations were made, but Mars is small, and very little surface detail could be discerned. Throughout the 18th and 19th centuries telescopes improved, revealing many dark areas on the red tinted surface. During the close opposition of 1877 sufficient detail could be seen that enabled Giovanni Schiaparelli to announce that he could see about 40 canali on Mars. This led to the saga of the canals of Mars, finally laid to rest in 1971 when Mariner 9 made observations from martian orbit showing that the canali/canals do not exist. Belief that there was life on Mars was widespread in the nineteenth century. However, the majority of astronomers never believed in martian intelligence. Least controversial was the view that the dark areas were some form of plant life. This view persisted until Mariner 4 flew past Mars in 1965 and discovered a far thinner atmosphere than previously thought. This was a low point, with impact craters dominating the images. It was Mariner 9 that revealed much more promising landscapes, including volcanic features, and others indicating that water had flowed across the surface, particularly when Mars was young. Thus, the contemporary era of Mars exploration began. Our picture of Mars today is not only much more complete than that before Mariner 4, in several ways it is quite different. But the belief that there might be life on Mars persists – subsurface life cannot be ruled out, and failing that, there might be ancient fossils on Mars. Key words: astrobiology, extraterrestrial life, history of astronomy, Mars. Mars – our current knowledge Before I outline what was known about Mars before the flyby of Mariner 4 in 1965, here is a very brief summary of our current knowledge of Mars, with an emphasis on features relevant to the possibility of martian life. IJA Mars pre space age + Figs BWJ Version 05Nov2008 2 of 28 13/11/2008 19:30 UT Mars is a small world, 53% the diameter of the Earth. Like the Earth it consists mainly of an iron rich core overlain by a silicate rich mantle, topped by a thin crust rich in silicates and in more volatile materials including water. Its orbit has a semimajor axis of 1.524 AU and an eccentricity of 0.0936. For the Earth these values are 1.000 AU (by definition) and 0.0167 respectively. Mars is our neighbour, being the next planet out from the Sun. Mars has a thin atmosphere consisting largely of CO2, with a column mass of only 0.015 × 104 kg m–2 (for the Earth the value is 1.07 × 104 kg m–2). This thin atmosphere, the sparse cloud cover, and the distance of Mars from the Sun, have made it a cold world. On a really good day in the tropics the temperature can reach up to around 20 °C, but it plunges to –100 °C or even lower at night. The surface has a reddish tint strewn with darker areas (Figure 1). It divides into two roughly equal areas, divided by a line inclined at nearly 40° with respect to the Equator. The northerly hemisphere shows considerable evidence of geological activity, perhaps confined to the past, and a corresponding low density of impact craters. The southerly hemisphere, being older, is much more impact scarred, and displays little evidence of geological activity. It does, however, bear features that seem to have been carved by liquid water (e.g. the channels in Figure 13), that suggest Mars was probably a much warmer, wetter place early in the first billion years or so of its 4.6 billion year history. Today, water has only been seen in solid form, in the residual north polar cap, in frosts that form at night, and as thin clouds of ice crystals, though it is probably present as liquid in places beneath the surface. Evidence for such subsurface liquid water is provided by narrow gulleys on slopes, some of which seem to have been carved in the last few years (Figure 2). Figure 1 Hubble Space Telescope images of the whole surface of Mars, in August 2003 when the planet was particularly close to the Earth. The white region at the bottom of each image is the residual south polar cap (CO2 frost and snow). The upward pointing dark triangular feature in the left image is Syrtis Major. (NASA/STScI, J Bell & M Wolff) Figure 2 Gullies in the south facing wall of the channel Nirgal Vallis, imaged by Mars Global Surveyor in 2000. Frame width 2.3 km. (NASA/JPL/Malin Space Science Systems) CO2, also condenses to form clouds and haze. It also condenses on to the surface, and accounts for the spread of each polar cap in that hemisphere’s winter – Mars’s axial inclination is similar to that IJA Mars pre space age + Figs BWJ Version 05Nov2008 3 of 28 13/11/2008 19:30 UT of the Earth (25.2° and 23.4° respectively), and so both planets suffer comparable seasonal changes in insolation, though as the martian “year” is 1.88 of our years, each season lasts considerably longer than on Earth. This is a very brief outline of our knowledge of Mars today. What about the past, and in particular before the advent in 1965 of the exploration of Mars by spacecraft? Mars before telescope observations Every 778 days on average, Mars, as seen from the Earth, moves to lie in the opposite direction from the Sun. Around such oppositions it becomes one of the brightest objects in the night sky. The regular motion among the stars of such a bright object must have drawn the attention of our ancestors well before they could record what they saw. From the dawn of written history a few millennia BC, Mars, and the four other visible planets that move among the stars (Mercury, Venus, Jupiter, and Saturn), were considered to be gods by many cultures. For example, the Greek name for Mars is Ares, the god of savage war, or bloodlust, fitting for the red tint of Mars, like a drop of blood in the sky. The Romans also associated the planet with war – Mars is the Roman god of war. Over the centuries many observations were made of the regular variation of the position of Mars with respect to the background of stars. Of great consequence is the particularly accurate observations made between 1576 and 1597 by the Danish nobleman and astronomer Tycho Brahe (1546-1601, Figure 3). His young assistant the German astronomer Johannes Kepler (1571-1630, Figure 3), who joined him in 1600, spent a long time trying to understand the path of Mars, and in 1609 he announced his first two laws of planetary motion. First law Each planet moves around the Sun in an ellipse, with the Sun at one focus, the other being empty. Second law The line from the planet to the Sun sweeps out equal areas in equal times. Figure 4(a) shows an orbit with a much greater eccentricity than that of Mars, to make Kepler’s laws pictorially clear. Figure 4(b) shows the orbit of Mars, with that of the Earth for comparison. It was Mars that led Kepler to his first two laws, because though Mercury’s orbit has an even greater eccentricity than that of Mars, it orbits much closer to the Sun than the Earth and so is impossible to observe over much of its orbit. Venus, Jupiter, and Saturn have much less eccentric orbits, and so departures from circular form are harder to discern. Additionally, Jupiter and Saturn have much IJA Mars pre space age + Figs BWJ Version 05Nov2008 4 of 28 13/11/2008 19:30 UT longer orbital periods than Mars, so have to be observed for much longer to cover a complete orbit. (Kepler’s third law also made use of Mars’s orbit, and states that the square of the sidereal period of a planetary orbit divided by the cube of the semimajor axis has the same value for all the planets in the Solar System.) Figure 3 Monument in Prague to Tycho Brahe (left) and Johannes Kepler (right). Figure 4 (a) An eccentric ellipse, which illustrates Kepler’s first two laws of planetary motion. The semimajor axis of the orbit is a and its eccentricity e. The shaded areas are equal and are swept out in equal times. (b) The orbits of Mars and the Earth. The point p on each orbit is the position of perihelion (the point closest to the Sun). The point a on the Earth’s orbit is the position of aphelion (the point furthest from the Sun). Kepler’s laws were a change in paradigm.
Details
-
File Typepdf
-
Upload Time-
-
Content LanguagesEnglish
-
Upload UserAnonymous/Not logged-in
-
File Pages28 Page
-
File Size-