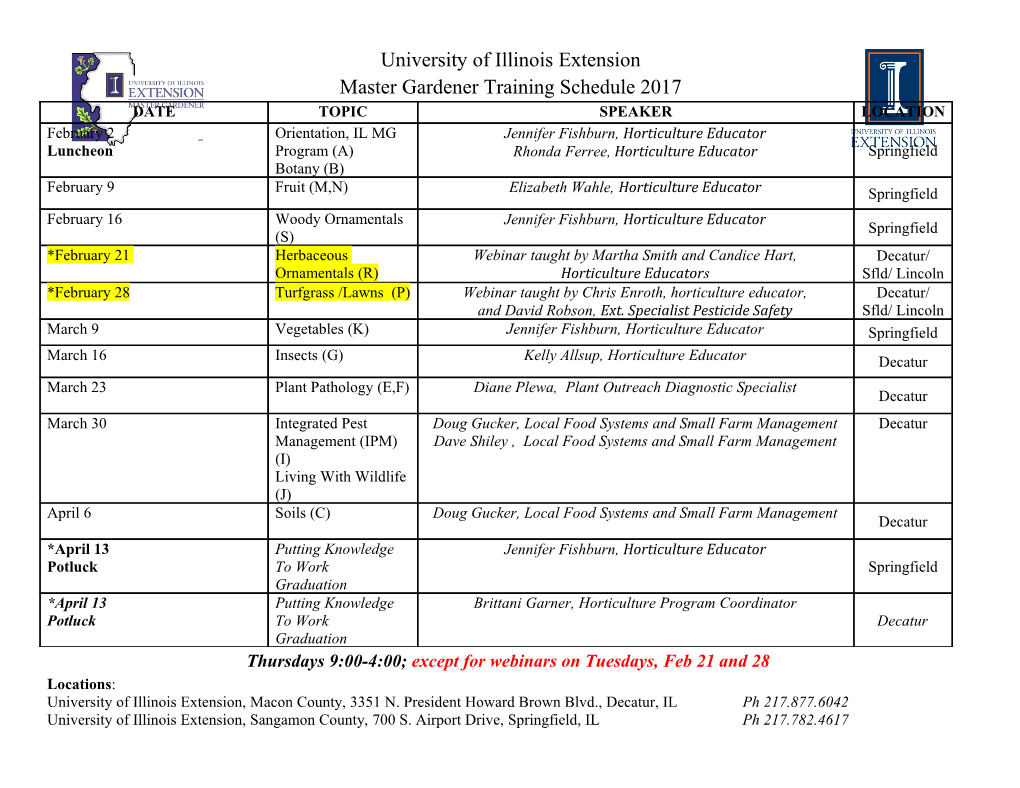
TSpace Research Repository tspace.library.utoronto.ca Review of Manganese Processing for Production of TRIP/TWIP Steels, Part 1: Current Practice and Processing Fundamentals Richart Elliott, Kenneth S. Coley, Sina Mostaghel, and Mansoor Barati Version Post-print/Accepted Manuscript Citation Elliott, R., Coley, K., Mostaghel, S. et al. JOM (2018) 70: 680. (published version) https://doi.org/10.1007/s11837-018-2769-4 Publisher’s statement This is a post-peer-review, pre-copyedit version of an article published in The Journal of The Minerals, Metals & Materials Society. The final authenticated version is available online at: http://dx.doi.org/10.1007/s11837-018-2769-4 How to cite TSpace items Always cite the published version, so the author(s) will receive recognition through services that track citation counts, e.g. Scopus. If you need to cite the page number of the author manuscript from TSpace because you cannot access the published version, then cite the TSpace version in addition to the published version using the permanent URI (handle) found on the record page. This article was made openly accessible by U of T Faculty. Please tell us how this access benefits you. Your story matters. A Review of Manganese Processing for the Production of TRIP/TWIP Steels Part 1: Current Practice and Processing Fundamentals R. Elliott1*, K. Coley1,2, S. Mostaghel3, and M. Barati1 1Department of Materials Science & Engineering, University of Toronto, Toronto, Canada 2McMaster Steel Research Centre, Department of Materials Science and Engineering, McMaster University, Hamilton, Canada 3Hatch Ltd., 2800 Speakman Drive, Mississauga, Canada *Contact: [email protected] Abstract The increasing demand for high performance steel alloys has led to the development of TRansformation Induced Plasticity (TRIP) and TWinning Induced Plasticity (TWIP) alloys over the past three decades. These alloys offer exceptional combinations of high tensile strength and ductility. Thus, the mechanical behaviour of these alloys has been a subject of significant work in recent years. However, the challenge of economically providing Mn in the quantity and purity required by these alloys has received considerably less attention. To enable commercial implementation of ultra high Mn alloys it is desirable to lower the high material costs associated with their production. Therefore, the present work reviews Mn processing routes in the context of the chemical requirements of these alloys. The aim of this review is to assess the current state-of-the-art regarding the reduction of manganese ores and provide a comprehensive reference for researchers working to mitigate the material processing costs associated with Mn production. The review is presented in two parts: Part 1 introduces TRIP and TWIP alloys, current industrial practice and pertinent thermodynamic fundamentals; Part 2 addresses the available literature regarding the reduction of Mn ores and oxides, and seeks to identify opportunities for future process development. 1 1. Introduction Manganese is the third most common constituent of steel. Annual global production of metallic manganese exceeded 13 million tonnes in 2013, with over 90% of mined manganese ores consumed by steelmaking. The remainder is used in applications such as batteries, fertilizers, catalysts, pigments and non-ferrous alloys [1–3]. Manganese is used in steelmaking as both a deoxidizer and an alloying agent [4]. Most steel grades (i.e. carbon and construction steels) average <1% Mn whereas current high manganese steel grades (i.e. Hadfield and 200-series stainless steels) contain 11-13% Mn. Despite accounting for <3% of the 1.6 billion tonnes of steel produced annually, these steels consume 20% of all annual manganese production [1,5]. TRansformation Induced Plasticity (TRIP) and TWinning Induced Plasticity (TWIP) steels also require an Mn content (4-30%) that is significantly higher than that required by most commercial alloys. However, the mechanical properties of these alloys makes them attractive for a range of applications, including the light-weighting of automobiles [6]. Widespread utilization of these steel grades will significantly increase the global demand for manganese alloying materials. The properties of these TRIP/TWIP steels and the challenges involved in their fabrication and forming have been, and continue to be, discussed at length. The cost of producing the required manganese units, however, has received considerably less attention. The present work is Part 1 of a two-part review of Mn processing in the context of ultra high manganese alloys. This portion of the review addresses the properties and chemistry of TRIP and TWIP alloys, the composition of Mn ores, the current state of commercial Mn processing, and the fundamental thermodynamics of Mn ore reduction. Part 2 will review the available literature regarding experimental reduction studies performed on Mn ores and oxides, and assess opportunities for new process development. The objective of this review is to assist in the design of a more energy efficient and economical processing strategy for high Mn alloys. 1.1 TRIP and TWIP Steels The behaviour of TRIP and TWIP steels is an active area of research and has been reviewed regularly and thoroughly over the past decade [6–16]. These reviews discuss at length the relationship between material properties and chemical composition of various high manganese alloys, typically with a focus on the ultimate tensile strength and total uniform elongation. To enable a discussion of the material processing requirements for these alloys, some relevant key concepts are outlined here. Figure 1 summarizes the tensile strength and ductility characteristic of most commercial steel types, including TRIP and TWIP. The Advanced High Strength Steels (AHSS) are divided into three generations. The 1st generation (<25 GPa%) encompasses a range of low Mn (typically <3%) containing alloys, characterized by their high tensile strength and comparatively low ductility. This includes a low-alloy TRIP or TRIP-assisted classification. The 2nd generation (>50 GPa%) is composed of high Mn (>10%) TRIP and TWIP alloys with exceptional ductility and high tensile strength. The 3rd generation (>35 GPa%) represents a compromise between cost and performance, sacrificing elongation and tensile strength for reduced material costs and manufacturability [15,17]. 2 Figure 1 General range of tensile strength (MPa) and total uniform elongation (%) possible within a variety of steel alloys. Alloys listed include: Interstitial Free (IF), Interstitial Free High Strength (IF-HS), Bake Hardening (BH), Carbon-Manganese (CMn), High Strength Low Alloy (HSLA), Dual Phase (DP), Complex Phase (CP), TRIP, Martensitic (MART), Quenching & Partitioning (Q&P), and TWIP. Adapted from Bouaziz et al. (2011), Keeler and Kimchi (2014), Lee and Han (2015), and Hernandez et al. (2016) [7,15,18,19]. The exceptional strength and ductility of TRIP and TWIP steels are the result of the deformation mechanisms for which they are named. In TRIP alloys, retained austenite undergoes a transformation to ε-martensite under strain [8]. In TWIP alloys, the application of strain induces the formation of mechanical twins, strengthening the alloy through a ‘dynamical Hall-Petch effect’; as the average grain size is decreased, the strength of the alloy correspondingly increases [12,20]. In both cases plasticity is maintained even at high strain. The deformation mechanism of an alloy correlates with its Mn content. TRIP behaviour is typically reported in steels with Mn content of 1-10%, while TWIP is reported in steels with >10% Mn. However, high Mn content alone is insufficient to produce TRIP or TWIP behaviour: an Fe-30Mn alloy displayed neither phenomena, despite its exceptionally high manganese content [7]. The deformation mechanism of an alloy also correlates with its Stacking Fault Energy (SFE), a parameter which characterises the energy required to create a defect in a material [21]. In high Mn steels, an SFE 3 <20 mJ/m2 favours TRIP while a SFE of 20-50 mJ/m2 favours TWIP. An SFE >50 mJ/m2 suppresses the formation of mechanical twins and the material will instead deform by slip [12,21,22]. To achieve the desired SFE, and thereby the desired mechanical performance, alloying elements may be added to the steel. Additions of Al, Si and N have all been reported to increase the SFE, while C additions result in a decreased SFE [8]. The effect of each wt-% alloy addition on the SFE per wt-% is typically 2 2 reported in the range of ±1-3mJ/m , although higher values on the order of ±5-10mJ/m have been reported [12]. The composition of TRIP/TWIP steels may also include additional elements such as Ni, Cr, Mo, and V which act to stabilize the retained austenite [23–25]. Typical compositional ranges for both TRIP and TWIP alloys are given in Table 1. Both alloys require tight control over their SFE, and therefore over their composition, in order to achieve the desired mechanical performance. While the maximum Mn concentration for a TRIP alloy is listed as 2.5% in Table 1, many alloys with higher Mn concentrations (i.e. 3rd generation AHSS) may still exhibit TRIP behaviour. The dependence of TRIP and TWIP behaviour on low concentrations of alloying elements, including tramp elements, represents one of the major challenges limiting their commercialization. In particular, it is costly to achieve the required Mn content in the alloy while maintaining sufficiently low C levels. Table 1 Typical range of major alloying elements for TRIP and TWIP steels [8,13,22,26–42]. Alloy Mn (%) Al (%) Si (%) C (%) Fe (%) TRIP 0.2-2.5 0-2 0.04-2.5 0.09-0.4 bal. TWIP 15-25 2-4 2-4 0.15-0.6 bal. The complex chemical composition of TRIP/TWIP alloys also complicates the fabrication and forming of these steels. In particular, it has been noted that the high strength (even at elevated temperatures), tendency to form non-metallic inclusions and banded structures, scale formation, and wide brittle-temperature range of these alloys present challenges in cold and hot-rolling due to the formation of cracks and surface defects [43–46].
Details
-
File Typepdf
-
Upload Time-
-
Content LanguagesEnglish
-
Upload UserAnonymous/Not logged-in
-
File Pages20 Page
-
File Size-