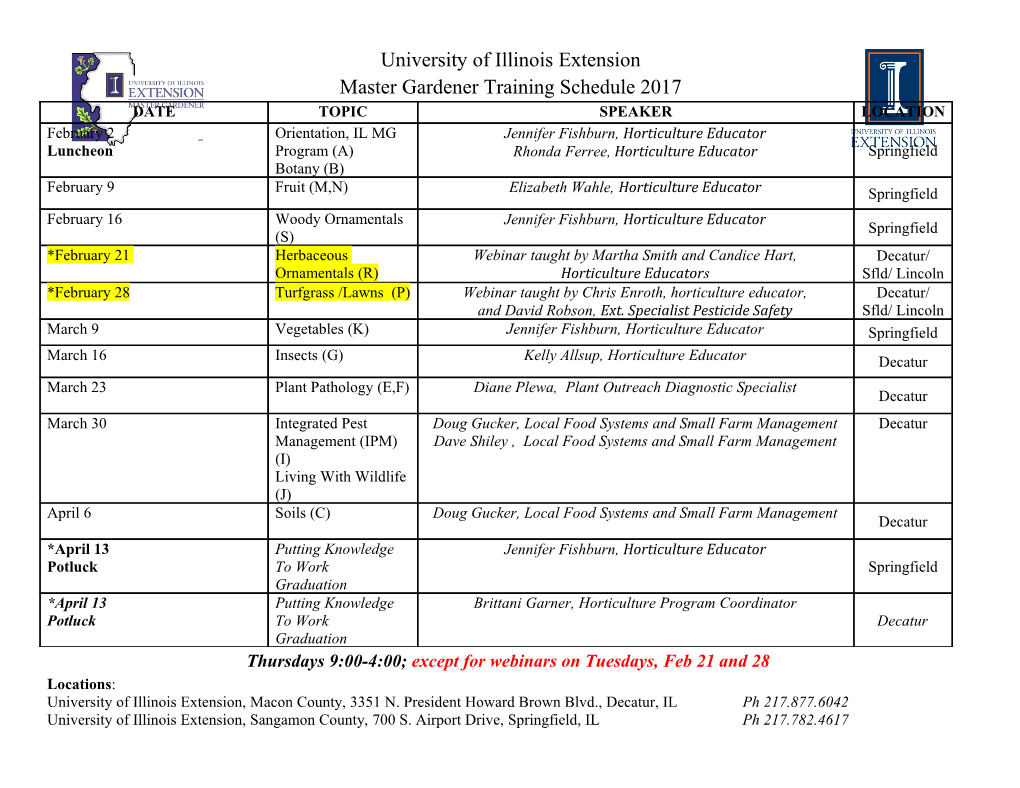
Environmental Microbiology Reports (2020) 12(1), 3–15 doi:10.1111/1758-2229.12787 Minireview Microbial degradation of phthalates: biochemistry and environmental implications Matthias Boll, 1* Robin Geiger,1 Madan Junghare2 Introduction and Bernhard Schink2 Phthalic acids (benzene dicarboxylic acids; phthalates) 1Faculty of Biology, Microbiology, University of Freiburg, consist of a benzene ring to which two carboxylic groups Freiburg, Germany. are attached. Depending on the position of the carboxylic 2Department of Biology and Microbial Ecology, groups to each other, three isomers are possible, namely University of Konstanz, Constance, Germany. phthalic acid (PA; ortho-positioned), isophthalic acid (IPA; meta-positioned) and terephthalic acid (TPA; para-posi- Summary tioned). In colloquial usage, the term ‘phthalate’ refers mostoftentotheortho isomer and is also used for its The environmentally relevant xenobiotic esters of esters. Phthalic acids are essential constituents of synthetic phthalic acid (PA), isophthalic acid (IPA) and ter- organic polymers that are commonly called plastics. In ephthalic acid (TPA) are produced on a million ton scale these materials, phthalates are either part of the polymeric annually and are predominantly used as plastic poly- structure as in poly(ethylene terephthalate) (PET), or mers or plasticizers. Degradation by microorganisms is they are non-covalently dissolved as low-molecular-weight considered as the most effective means of their elimina- di-esters within the polymeric structure to act as softeners tion from the environment and proceeds via hydrolysis or plasticizers. to the corresponding PA isomers and alcohols under Plastic materials are used in almost every aspect of mod- oxic and anoxic conditions. Further degradation of PA, ern life and offer solutions and features that other materials IPA and TPA differs fundamentally between anaerobic were not able to provide before (Andrady and Neal, 2009). and aerobic microorganisms. The latter introduce Due to their low production costs and lack of cheap alterna- hydroxyl functionalities by dioxygenases to facilitate tives, there is an increasing demand of synthetic organic subsequent decarboxylation by either aromatizing polymers worldwide (Thompson et al., 2009). For instance, dehydrogenases or cofactor-free decarboxylases. In contrast, anaerobic bacteria activate the PA isomers to the packaging industry alone accounts for approximately fi the respective thioestersusingCoAligasesorCoA 42% of the global use of all non- bre plastics, followed by transferases followed by decarboxylationtothecentral the building and construction sector accountable for about fi intermediate benzoyl-CoA. Decarboxylases acting on 19% of non- bre plastics (Geyer et al., 2017). It is estimated the three PA CoA thioesters belong to the UbiD enzyme that the worldwide annual production of plastics will double family that harbour a prenylated flavin mononucleotide to 600 million tons within 20 years (Kumar, 2018). Despite (FMN) cofactor to achieve the mechanistically challeng- their numerous advantages, plastics cause increasing envi- ing decarboxylation. Capture of the extremely instable ronmental problems and health concerns worldwide PA-CoA intermediate is accomplished by a massive (Thompson et al., 2009). Some of these problems start only overproduction of phthaloyl-CoA decarboxylase and a at the end of their designated purpose when plastics balanced production of PA-CoA forming/decarboxylating become waste. Europe disposes approximately 50% of its fi enzymes. The strategy of anaerobic phthalate degrada- plastic waste in engineered land lls, while in other parts of tion probably represents a snapshot of an ongoing the world it is still incinerated openly, emitting large amount evolution of a xenobiotic degradation pathway via a of toxic or greenhouse gases. Huge freights of plastic mate- short-lived reaction intermediate. rials end up in the oceans where they persist for long times and endanger birds and other marine animals. Although recycling might be an option to limit production of new plas- tics, this material primarily ends up in secondary products *For correspondence. E-mail [email protected]; with less demanding material properties and unclear fate, Tel. (+49) 761 2032649; Fax (+49) 761 2032626. and is hence not a permanent solution. Furthermore, © 2019 The Authors. Environmental Microbiology Reports published by Society for Applied Microbiology and John Wiley & Sons Ltd. This is an open access article under the terms of the Creative Commons Attribution License, which permits use, distribution and reproduction in any medium, provided the original work is properly cited. 4 M. Boll, R. Geiger, M. Junghare and B. Schink recycling will not prevent the rise of microplastics in marine environments which is a growing problem that is difficult to solve (Hahladakis et al., 2018). Therefore, it is important to develop new strategies of waste management and to better understand plastic biodegradation as a sustainable per- spective for removal of plastics in nature. Phthalic acid ester use and biodegradation Phthalic acid polyesters. Phthalates can form essential build- ing blocks inside plastic polymers (1). In these cases, phthalates (most often TPA) are bound via ester linkages to divalent alcohols such as ethylene glycol, 1,4-butanediol, 1,6-hexanediol, and so on. Needless to say, these covalently linked TPA residues are rather difficult to release from their polymeric mother backbones, as this is true for synthetic poly- mers in general. Although ester bonds are comparably easy to cleave by hydrolysis and require only water as a co-substrate, degradation of such polymers is difficult because a hydrolytic enzyme first has to get access to such polymer fibres, and this would require swelling of the plastic material by excessive water uptake. Plastics are typically produced as water-resis- tant, rather hydrophobic structures that are hardly accessible Fig. 1. Examples of industrially produced esters of the three phthalic to hydrolytic enzymes. Moreover, hydrolytic enzymes pro- acid isomers. A. Polyethylenglycol terephthalate ester polymer (PET). duced by microbes are typically synthesized rather specifically B. Polyethylenglycol IPA esters used as co-polymer of PET. C. PA esters used as plasticizers, for typical alcohols residues (=R) see Table 1. for attack on specific ester structures and do not interact freely with novel ester linkages. Last but not least, synthesis of extra- cellular enzymes for polymer degradation is an expensive covers in agriculture, or for plastic shopping bags. The BASF fi ® effort for a microbial cell and has to guarantee a suf cient pay- company produces Ecoflex , a copolymer of 1,4-butanediol back by easily degradable breakdown products that can cover with alternating terephthalate and adipate (hexane dioic acid) the energy expenditure for enzyme synthesis on the side of residues (poly butylene-adipate-terephthalate; PBAT). The ‘ the producer. Thus, the breakdown products should be palat- material decomposes in compost and agricultural soil within ’ able and should easily provide metabolic energy for a broad several months and thus fulfils its task to survive one vegeta- community of microbes of which then only few would dare to tion period and to disappear before the next one starts. In invest into the development of suited enzymes for polymer our lab, we could confirm that this material decomposes hydrolysis. As we will see later, the biochemistry of phthalate under oxic conditions and also anaerobically in the presence degradation is a rather demanding task, and the aromatic of nitrate, although nitrate-dependent degradation turned out derivatives formed in its breakdown are energetically not too to be far slower with terephthalate-containing polymers than rewarding to justify every effort into such an enterprise. Poly- with adipate-containing ones. There was no significant deg- saccharides, proteins, nucleic acids and so on are much more radation of either type of polymer in incubations under strictly appealing in this respect. This is true also for the natural poly- anoxic conditions with either sulfate or CO2 as the only elec- esters, e.g., poly-3-hydroxybutyric acid, a bacterial storage tron acceptor (Schink, unpublished). This is surprising as polymer which is easily degradable by a broad community of hydrolytic depolymerization of such synthetic material should aerobic and anaerobic bacteria (Jendrossek and Handrick, be possible under any condition, independent of the avail- 2002; Hazer and Steinbüchel, 2007). able electron acceptor. We assume that the probability of PET (Fig. 1A) is produced at high rate (56 million tons in development of efficient polymer-degrading hydrolases may 2013; Kawai et al., 2019), mainly as containers for soft drinks be higher in the aerobic and nitrate-dependent microbial and so on. Its microbial degradation is very slow and incom- world than among the strict anaerobes. Moreover, production plete. It appears that only surface-exposed residues are of extracellular hydrolases for unusual polymeric substrates degraded by microbes to a limited extent through the action of may be too ‘expensive’ for strict anaerobes with their limited cutinases, lipases and other carboxylesterases (Kawai et al., energy budgets. We have to conclude that phthalate resi- 2019). As this plastic material is applied mainly as drink con- dues that are covalently bound inside polymeric plastic tainers it has little tendency
Details
-
File Typepdf
-
Upload Time-
-
Content LanguagesEnglish
-
Upload UserAnonymous/Not logged-in
-
File Pages13 Page
-
File Size-