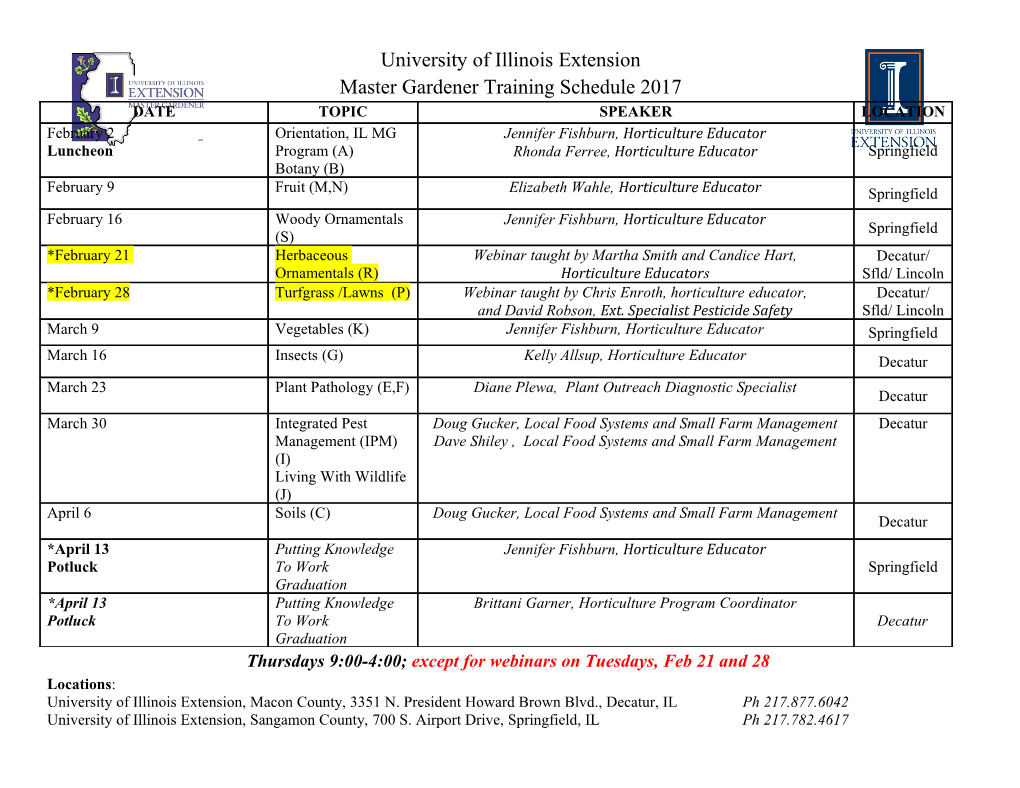
INVESTIGATION OF THE ELECTROPHYSIOLOGICAL PROPERTIES OF THE MAJOR CELL TYPES IN THE RAT OLFACTORY TUBERCLE By ELIZABETH CHEN CHIANG Submitted in partial fulfillment of the requirement For the degree Doctor of Philosophy Thesis Advisor: Dr. Ben W. Strowbridge Department of Neurosciences CASE WESTERN RESERVE UNIVERSITY January, 2008 Case Western Reserve University School of Graduate Studies We hereby approve the thesis/dissertation of Elizabeth Chen Chiang candidate for the PHD degree*. signed Jerry Silver Chair of the Committee Ben Strowbridge Gary Landreth Ruth Siegel Lynn Landmesser October 5, 2007 *We also certify that written approval has been obtained for any proprietary material contained within. ii To my parents, Dalen and Ru-fang Chiang, for teaching me the value of learning and education from a young age and always supporting my academic pursuits iii Table of Contents Title Page…………………………………………………………………………………..i Committee Signatures……………………………………………………………………..ii Dedication………………………………………………………………………………...iii Table of Contents…………………………………………………………………………iv List of Tables…………………………………………………………………………......vi List of Figures…………………………………………………………………………....vii Acknowledgements……………………………………………………………………......x List of Abbreviations…………………………………………………………………..…xi Abstract……………………………………………………………………………….…xiv Chapter 1: Introduction……………………………………………………………………1 Basic description of the olfactory system…………………………………………2 Structure of the olfactory tubercle………………………………………………...4 Potential circuitry of the olfactory tubercle………………………………………7 The olfactory tubercle as part of the ventral striatum……………………………13 Chapter 2: Diversity of neural signals mediated by multiple burst-firing mechanisms in rat olfactory tubercle neurons……………………………………………………………36 Introduction………………………………………………………………………37 Methods…………………………………………………………………………..39 Results……………………………………………………………………………43 Discussion………………………………………………………………………..54 Chapter 3: Simulation of the principle currents of the nonregenerative bursting cell of the rat olfactory tubercle……………………………………………………………………102 Introduction……………………………………………………………………..103 Methods and Results……………………………………………………………105 iv Discussion………………………………………………………………………128 Chapter 4: Discussion…………………………………………………………………..177 Two mechanisms of generating burst firing……………………………………178 Role of calcium in bursting cells…………………………………………….…179 Comparing the tubercle bursting cell to the hippocampal bursting cell……..…183 Function of bursting activity and plasticity………………………………….…185 The tubercle as part of the ventral striatum………………………………….…186 Circuitry of the olfactory tubercle……………...……………………………….187 The tubercle as a site of action for anti-psychotics and cocaine addiction……..191 The tubercle is a sensory, limbic, and motor structure…………………………194 Chapter 5: Future Directions…………………………………………………………...196 Circuitry of the olfactory tubercle……………………………………………....197 Role for bursting cells………………………………………………………..…199 Function of dopamine receptors and cocaine in the tubercle………………..….201 Conclusion…………………………………………………………………...…207 Chapter 6: Bibliography………………………………………………………………...213 v List of Tables Table 2-1 Membrane properties of tubercle neurons…………………………………….61 Table 5-1 Summary of results from Luskin and Price (1983) regarding the olfactory tubercle…………………………………………………………………………………208 vi List of Figures Figure 1-1 Schematic diagram showing the ventral structures contained in tubercle slices………………………………………………………………………….18 Figure 1-2 Nissl stain of corona slice of rat olfactory tubercle…………………………..20 Figure 1-3 Coronal slices of rat brain stained for proteins related to neurotransmitter glutamate……………………………………………………………………..22 Figure 1-4 Regional distribution of VGLUT3 mRNA and protein……………………...24 Figure 1-5 Immunohistochemical localization of VGLUT3 on a coronal rat brain section taken at the level of the tubercle and the accumbens………………………...27 Figure 1-6 Coronal slice of the rat brained stained with acetylcholinesterase…………..29 Figure 1-7 Diagram of ChAT+ and GAD+ neurons in the tubercle……………………..32 Figure 1-8 Comparison of the distribution of D1 (A), D2, (B), and D3(C) mRNA in the rat brain……………………………………………………………………....34 Figure 2-1 Schematic diagram showing the ventral structures contained in tubercle slices……………………………………………………………….…………62 Figure 2-2 Graded responses in three types of olfactory tubercle neurons………………64 Figure 2-3 Morphology of regular spiking tubercle neurons…………………………….66 Figure 2-4 Morphology of intermittently discharging and bursting tubercle neurons…...68 Figure 2-5 Correlations of burst properties with stimulus strength……………………...70 Figure 2-6 Quantitative analysis of burst properties……………………………………..72 Figure 2-7 Bursting cell response to depolarizing ramp stimuli…………………………74 Figure 2-8 Cs increases firing during depolarizing steps in bursting cells………………76 Figure 2-9 Low Ca ACSF increases firing in bursting cells……………………………..78 Figure 2-10 TTX does not block depolarizing plateau potentials………………………..80 Figure 2-11 Reduction of input resistance during afterhyperpolarization of bursting cells…………………………………………………………………………..82 vii Figure 2-12 Comparison of response of regenerative and nonregenerative bursting cells…………………………………..…………………………………..…..84 Figure 2-13 Differences in burst properties between regenerative and nonregenerative bursting cells…………………………………………………………………86 Figure 2-14 Plateau potential evoked by graded steps…………………………………..88 Figure 2-15 Membrane potential of plateaus are constant………………………………90 Figure 2-16 Plateau potentials seen with brief stimuli………………………………..…92 Figure 2-17 Low Ca abolishes plateau potentials…………………………………….….94 Figure 2-18 Plasticity of burst responses in nonregenerative cells………………………96 Figure 2-19 Brief depolarizing steps suppresses later activity…………………………..98 Figure 2-20 Responses of tubercle neurons to sniffing-like periodic input………….…100 Figure 3-1 Morphology of bursting tubercle neuron…………………………………...133 Figure 3-2 Figure 3-2: Montage of 2-photon images of a nonregenerative bursting neuron in the MFL………………………………………………………………….135 Figure 3-3 Schematic diagram of morphology of neuron model……………………….137 Figure 3-4 Correlations of burst properties with stimulus strength………………….…139 Figure 3-5 One mathematical model of IH with different distributions across model cell……………………………………………………………………….….141 Figure 3-6 Five different mathematical models of IH………………………………..…143 Figure 3-7 Comparison of three experimentally recorded cells with the simulation…...145 Figure 3-8 Comparison of the control simulation to the simulation after removal of IH.147 Figure 3-9 Different spatial distributions of T, L and N current in the model neuron…149 Figure 3-10 Variation in the distribution of T current with L and N current in the soma and dendrites……………………………………………………………..…151 Figure 3-11 Variation in the magnitude of T current……………………………….......153 Figure 3-12 Nonregenerative bursting cells at different membrane potentials…………155 Figure 3-13 Graded response of the simulation run in control conditions and run with the removal of calcium activated potassium current…………………………...157 viii Figure 3-14 Graded response of the simulation run in control conditions and run with the removal of M current…………………………………………………….…159 Figure 3-15 Effect of a low calcium environment on the model cell………………..…161 Figure 3-16 Response of model to simulated condition of the addition of TTX…….....163 Figure 3-17 Comparison of the effects of TTX on recorded cells vs. the simulated model cell…………………………………………………………………………..165 Figure 3-18 Response of model to TTX compared to TTX and Low Ca ACSF……….167 Figure 3-19 Response of model to brief stimuli……………………………………..…169 Figure 3-20 2 Inhibition of firing following a long stimulus…………………………..171 Figure 3-21 Inhibition of firing following a brief stimulus…………………………….173 Figure 3-22 Loss of suppression of firing with loss of CaK current…………………...175 Figure 5-1 Diagram of neurons of a brain slice of the olfactory tubercle………………209 Figure 5-2 Hypothesized circuit of the olfactory tubercle……………………………...211 ix Acknowledgements I would like to thank my thesis advisor, Dr. Ben Strowbridge, for years of advice and guidance. I would also like to thank the members of my thesis committee for their helpful suggestions in my research. Lastly, I’d like to thank the past and present members of the Strowbridge lab for their assistance, discussions, and companionship. x List of Abbreviations ACSF: artificial cerebrospinal fluid AP: action potential 4-AP: 4-aminopyridine, fast K channel blocker BAPTA: O,O’-Bis(2-aminophenyl)ethyleneglycol-N,N,N’,N’-tetraacetic acid tetrapotassium salt cAMP: cyclic adenosine monophosphate ChAT: choline acetyltransferase DCL: Dense Cell Layer D: dopamine DNPI: differentiated-associated Na+ dependent inorganic phosphate cotransporter EGTA: O,O’-Bis(2-aminoethyl)ethyleneglycol-N,N,N’,N’-tetraacetic acid, slow Ca chelator EPSC: excitatory postsynaptic current EPSP: excitatory postsynaptic potential GABA: gamma-aminobutyric acid, the neurotransmitter in granule cells GAD: glutamic acid decarboxylase GC: granule cell HRP: horse radish peroxidase HVA: high voltage activated IC: Islands of Calleja IF: intermittently-firing xi IPSC: inhibitory postsynaptic current IPSP: inhibitory postsynaptic potential ISI: inter-spike interval LOT: lateral olfactory tract, axons of mitral cells LTD: long term depression LTP: long term potentiation LVA: low voltage activated MFL: multiform layer ML: molecular layer mRNA: messenger ribonucleic acid NBQX: 1,2,3,4-tetrahydro-6-nitro-2,3-dioxo-benzo[f]quinoxaline-7-sulfonamide,
Details
-
File Typepdf
-
Upload Time-
-
Content LanguagesEnglish
-
Upload UserAnonymous/Not logged-in
-
File Pages241 Page
-
File Size-