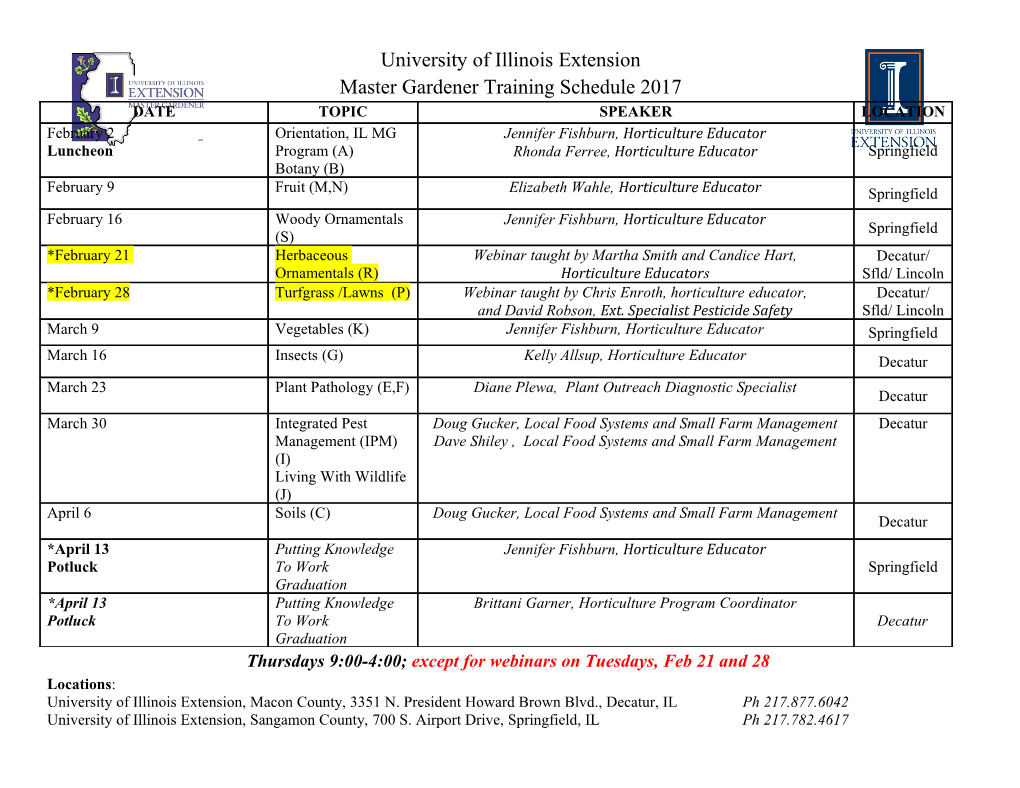
MA482 Stochastic Analysis Lectured by Prof. David Elworthy ([email protected]) Typed by Tim Sullivan¤([email protected]) University of Warwick Term 1, 2005{2006 Printed November 12, 2006 ¤Thanks to P.H.D. O'Callaghan for pointing out and correcting typographical errors. 1 Contents 1 Re-Cap of Measure Theory 4 2 Fourier Transforms of Measures 6 3 Gaussian Measures on Finite-Dimensional Spaces 8 3.1 Gaussian Measures . 8 3.2 Fourier Transforms of Gaussian Measures . 10 4 Gaussian Measures on Banach Spaces 12 5 Cylinder Set Measures 13 6 The Paley-Wiener Map and the Structure of Gaussian Measures 18 6.1 Construction of the Paley-Wiener Integral . 18 6.2 The Structure of Gaussian Measures . 21 7 The Cameron-Martin Formula: Quasi-Invariance of Gaussian Measures 23 8 Stochastic Processes and Brownian Motion in Rn 27 8.1 Stochastic Processes . 27 8.2 Construction of It¹o'sIntegral . 28 9 It¹oIntegrals as Divergences 40 9.1 The Clark-Ocone Theorem and Integral Representation . 40 9.2 Chaos Expansions . 46 References [BP] CzesÃlaw Bessaga & Alexsander PeÃlczy¶nski. Selected Topics in In¯nite-Dimensional Topology. Warszawa: PWN, 1975. [MX] Mao Xuerong. Stochastic Di®erential Equations and their Applications. Chichester: Horwood, 1997. [Â] Bernt Âksendal, Stochastic Di®erential Equations Sixth Edition. Berlin; New York: Springer, 2003. [RS] Michael Reed & Barry Simon. Methods of Modern Mathematical Physics. New York; London: Academic Press, 1980. [RW1] L.C.G. Rogers & David Williams. Di®usions, Markov Processes and Martingales. Vol. 1: Foundations. Chichester: Wiley, 1994. [RW2] L.C.G. Rogers & David Williams. Di®usions, Markov Processes and Martingales. Vol. 2: It¹oCalculus. Chichester: Wiley, 1994. 2 Introduction Stochastic analysis can be viewed as a combination of in¯nite-dimensional analysis, measure theory and linear analysis. We consider spaces such as the in¯nite-dimensional space of paths in a given state space. ² Around 1900, Norbert Wiener (1894{1964) introduced the notion of Wiener measure. This leads to ideas of \homogeneous chaos" and analysis of brain waves. R ² Richard Feynman (1918{1988) worked on quantum mechanics and quantum physics, using ideas like R paths in R3 and maps Rp!Rq . R ² Stephen Hawking (1942{) took this idea further: universes. ² Edward Witten (1951{) applied these methods to topology, topological invariants and knot theory. ² This area's connections with probability theory lend it the label \stochastic". Areas of interest include Brownian motion and other stochastic dynamical systems. A large area of application is mathematical ¯nance. This course is not on stochastic dynamical systems. 3 1 Re-Cap of Measure Theory De¯nitions 1.1. A measurable space is pair f­; F g where ­ is a set and F is a σ-algebra on ­, so ; 2 F , S1 A 2 F =) ­ n A 2 F and A1;A2; ¢ ¢ ¢ 2 F =) i=1 Ai 2 F . Example 1.2. Given a topological space X, the Borel σ-algebra B(X) is de¯ned to be the smallest σ-algebra containing all open sets in X. We will always use this unless otherwise stated. De¯nitions 1.3. If fX; A g and fY; Bg are measurable spaces, f : X ! Y is measurable if B 2 B =) f ¡1(B) 2 A . In general, σ(f) := ff ¡1(B)jB 2 Bg is a σ-algebra on X, the σ-algebra generated by f. It is the smallest σ-algebra on X such that f is measurable into fY; Bg. If X; Y are topological spaces then f : X ! Y continuous implies that f is measurable | this is not quite trivial. De¯nitions 1.4. A measure space is a triple f­; F ; ¹g with f­; F g a measure space and ¹ a measure on it, i.e. a S1 P1 ¹ : F ! R¸0 [ f1g such that ¹(;) = 0 and A1;A2; ¢ ¢ ¢ 2 F disjoint =) ¹( i=1 Ai) = i=1 ¹(Ai) · 1. The space has ¯nite measure (or ¹ is a ¯nite measure) if ¹(­) < 1. f­; F ; ¹g is a probability space (or ¹ is a probability measure) if ¹(­) = 1. De¯nition 1.5. Given a measure space f­; F ; ¹g, a measurable space fX; A g and f : ­ ! X measurable, de¯ne ¡1 the push-forward measure f¤¹ on fX; A g by (f¤¹)(A) := ¹(f (A)). As an exercise, check that this is indeed a measure on fX; A g. Exercises 1.6. (i) Check that f¤¹ is indeed a measure on fX; A g. (ii) Show that if f; g : ­ ! X are measurable and f = g ¹-almost everywhere, then f¤¹ = g¤¹. Examples 1.7. (i) Lebesgue measure ¸n on Rn: Borel measure such that ¸n(rectangle) = product of side lengths, e.g. ¸1([a; b]) = b ¡ a. This determines ¸n uniquely | see MA359 Measure Theory. n n n n n Take v 2 R . De¯ne Tv : R ! R by Tv(x) := x + v. Then (Tv)¤(¸ ) = ¸ since translations send rectangles to congruent rectangles and ¸n is unique. Thus ¸n is translation-invariant. (ii) Counting measure c on any fX; A g: c(A) := #A. Counting measure on Rn is also translation-invariant. (iii) Dirac measure on any fX; A g: given x 2 X, de¯ne ½ 1 x 2 A; ± (A) := x 0 x 62 A: n Dirac measure ±x on R is not translation-invariant. De¯nitions 1.8. Let X be a topological space (with its usual σ-algebra B(X)). A measure ¹ on X is locally ¯nite if for all x 2 X, there exists an open U ⊆ X with x 2 U and ¹(U) < 1. ¹ on X is called strictly positive if for all non-empty open U ⊆ X, ¹(U) > 0. Examples 1.9. (i) ¸n is locally ¯nite and strictly positive. (ii) c is not locally ¯nite in general, but is strictly positive on any X. (iii) ±x is ¯nite, and so locally ¯nite, but is not strictly positive in general. Proposition 1.10. Suppose that H is a (separable) Hilbert space with dim H = 1. Then there is no locally ¯nite translation invariant measure on H except ¹ ´ 0. (Therefore, there is no \Lebesgue measure" for in¯nite- dimensional Hilbert spaces.) Recall that ² a topological space X is separable if it has a countable dense subset, i.e. 9x1; x2; ¢ ¢ ¢ 2 X such that X = fx1; x2;::: g; ² if a metric space X is separable then for any open cover fU®g®2A of X there exists a countable subcover; 4 ² non-separable spaces include L(E; F ) := fcontinuous linear maps E ! F g when E; F are in¯nite-dimensional Banach spaces, and the space of HÄolder-continuous functions C0+®([0; 1]; R). Proof. Suppose that ¹ is locally ¯nite and translation invariant. Local ¯niteness implies that there is an open non-empty U such that ¹(U) < 1. Since U is open, there exist x 2 U and r0 > 0 such that Br0 (x) ⊆ U. Then ¹(Br0 (x)) < 1 as well. By translation, ¹(Br(y)) < 1 for all y 2 H and r · r0. Fix an r 2 (0; r0); S S1 then H = y2H Br(y), an open cover. By separability, there exist y1; y2; ¢ ¢ ¢ 2 H such that H = j=1 Br(yj), so ¹(Br(yj)) > 0 for some j, and so ¹(Br(y)) > 0 for all y 2 H and r > 0. Set c := ¹(Br0=30(y)) for any (i.e., all) y 2 H. Observe that if e1; e2;::: is an orthonormal basis for H then Br0=30(ej=2) ⊆ Br0 (0) for all j. By Pythagoras, P1 these balls are disjoint. ¹(Br0 (0)) ¸ j=1 c = 1 unless ¹ ´ 0. But ¹ 6´ 0 =) ¹(Br0 (0)) < 1 by local ¯niteness, a contradiction. De¯nition 1.11. Two measures ¹1; ¹2 on f­; F g are equivalent if ¹1(A) = 0 () ¹2(A) = 0. If so, write ¹1 ¼ ¹2. Example 1.12. Standard Gaussian measure γn on Rn: Z 2 γn(A) := (2¼)¡n=2 e¡kxk =2 dx A n n 2 2 2 n n ¡kxk2=2 for A 2 B(R ). Here dx = d¸ (x) and kxk = x1 + ¢ ¢ ¢ + xn for x = (x1; : : : ; xn). ¸ ¼ γ since e > 0 for all x 2 Rn De¯nition 1.13. Given a measure space f­; F ; ¹g, let f : ­ ! ­ be measurable. Then ¹ is quasi-invariant under ¡1 f if f¤¹ ¼ ¹, i.e. ¹(f (A)) = 0 () ¹(A) = 0 for all A 2 F . Example 1.14. γn is quasi-invariant under all translations of Rn. Theorem 1.15. If E is a separable Banach space and ¹ is a locally ¯nite Borel measure on E that is quasi-invariant under all translations then either dim E < 1 or ¹ ´ 0. The proof of this result is beyond the scope of this course, although it raises the question: are there any \interesting" and \useful" measures on in¯nte-dimensional spaces? 5 2 Fourier Transforms of Measures De¯nition 2.1. Let ¹ be a probability measure on a separable Banach space E. Let E¤ := L(E; R) be the dual space. The Fourier transform ¹^ : E¤ ! C is given by Z ¹^(`) := ei`(x) d¹(x) E p R R ¤ i`(x) ¤ for ` 2 E , where i = ¡1 2 C. It exists since E je j d¹(x) = E d¹(x) = ¹(E) = 1 < 1. In fact, for all ` 2 E , j¹^(`)j · 1 and¹ ^(0) = ¹(E) = 1. For E a Hilbert space H with inner product h¢; ¢i, the Riesz Representation Theorem gives an isomorphism H¤ ! H : ` 7! `], where `] 2 H has h`]; xi = `(x) for all x 2 H. Therefore, we can consider¹ ^] : H ! C given by Z ¹^](h) := eihh;xi d¹(x): H So¹ ^](`]) =¹ ^(`).
Details
-
File Typepdf
-
Upload Time-
-
Content LanguagesEnglish
-
Upload UserAnonymous/Not logged-in
-
File Pages47 Page
-
File Size-