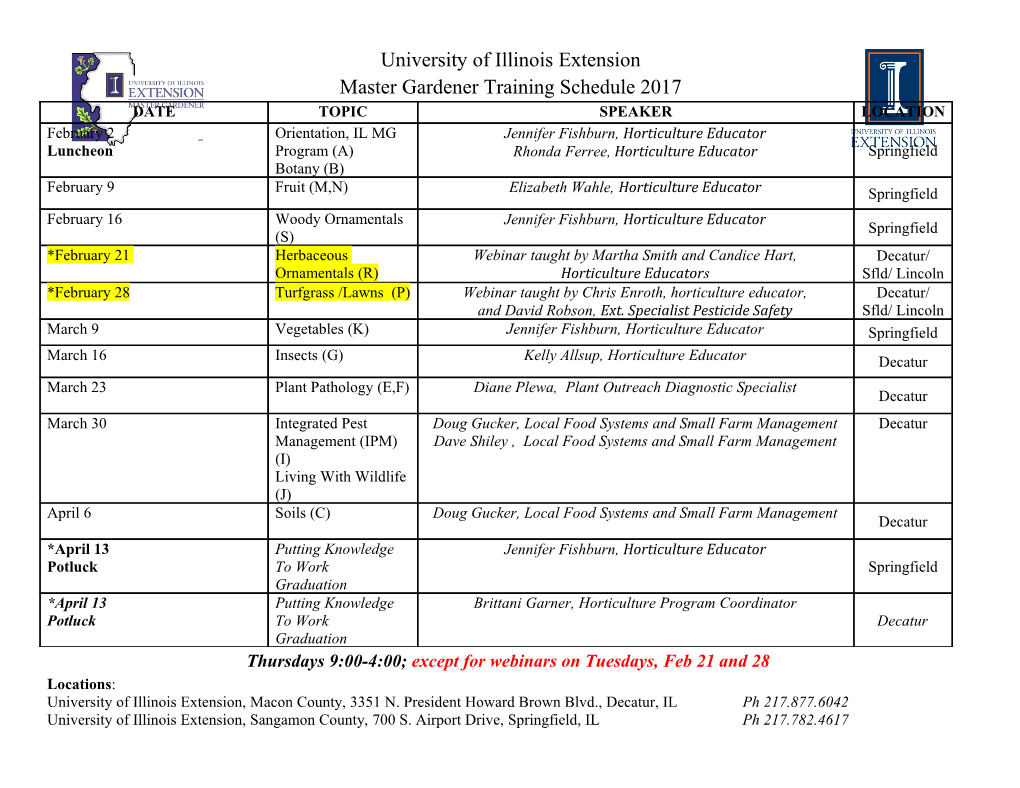
W&M ScholarWorks Undergraduate Honors Theses Theses, Dissertations, & Master Projects 5-2017 Structural Comparison of Copper(II) Thiocyanate Pyridine Complexes Joseph V. Handy College of WIlliam & Mary Follow this and additional works at: https://scholarworks.wm.edu/honorstheses Part of the Inorganic Chemistry Commons Recommended Citation Handy, Joseph V., "Structural Comparison of Copper(II) Thiocyanate Pyridine Complexes" (2017). Undergraduate Honors Theses. Paper 1100. https://scholarworks.wm.edu/honorstheses/1100 This Honors Thesis is brought to you for free and open access by the Theses, Dissertations, & Master Projects at W&M ScholarWorks. It has been accepted for inclusion in Undergraduate Honors Theses by an authorized administrator of W&M ScholarWorks. For more information, please contact [email protected]. Structural Comparison of Copper(II) Thiocyanate Pyridine Complexes A thesis submitted in partial fulfillment of the requirement for the degree of Bachelor of Science in Chemistry from The College of William & Mary by Joseph Viau Handy Accepted for ____________________________ ________________________________ Professor Robert D. Pike ________________________________ Professor Deborah C. Bebout ________________________________ Professor David F. Grandis ________________________________ Professor William R. McNamara Williamsburg, VA May 3, 2017 1 Table of Contents Table of Contents…………………………………………………...……………………………2 List of Figures, Tables, and Charts………………………………………...…………………...4 Acknowledgements….…………………...………………………………………………………6 Abstract……………………………….……………………………………………………...…...7 Introduction………………………………………………………………………………………8 Electronic Orbitals and Crystal Field Splitting in Copper(II)……………………………..8 Hard-Soft Lewis Acid-Base Theory…………………………………………………..…12 Metal-Organic Frameworks..…………………………………………………………….13 Thiocyanate Ligands……………………………………………………………………..15 Pyridine Ligands…………………………………………………………………………16 This Project………………………………………………………………………………18 Experimental……………………………………………………………………………………21 Materials…………………………………………………………………………………21 General Synthesis ……………………………………………….………………………21 Synthesis of trans-[Cu(SCN2(µ-XPy)] Crystals…………………………………………22 X-Ray Crystallography…………………………………………………………………..23 Atomic Absoprtion Analysis……………………………………………………………..23 Results and Discussion………………………………………………………………………….24 Overview…………………………………………………………………………………24 Synthesis…………………………………………………………………………………24 Elemental Analysis Results………………………………………………………………28 Infrared Spectroscopy Results…………………………………………………………...30 2 Crystallographic Results…………………………………………………………………33 X-Ray Crystal Structures…………………………………………………..…………….42 Discussion………………………………………………………………………………..61 Conclusion……………………………………………………………………………...……….65 References……………………………………………………………………………………….66 3 List of Figures, Tables, and Charts Figure 1: 3-D geometries of the five d-orbitals…………………………………………………..9 Figure 2: Common crystal field splitting of metal d-orbitals…………………………………...10 2+ Figure 3: Z-in and z-out Jahn-Teller distortion in Cu …………………………………………………………..11 Figure 4: Visual representation of the construction of MOFs…………………………………..14 Figure 5: Resonance forms and binding modes of thiocyanate…………………………………15 Figure 6: Pyridine and derivatives used in this study…………………………………………...17 Table 1: Synthesis results………………………………………………………………………..27 Table 2: Elemental analysis results……………………………………………………………...29 Table 3: Infrared spectroscopy data……………………………………………………………..32 Table 4: Crystallographic data for all compounds………………………………………………33 Figure 7: π-stacking interactions………………………………………………………………..41 Table 5: Selected bond lengths and angles for all compounds………………………………….42 Table 6: Complexes sorted by structural category………………………………………………46 Figure 8: X-ray structures of new D4h chain complexes………………………………………...49 Figure 9: X-ray structure of trans-[Cu(SCN)2(4-MePy)2]………………………………………50 Figure 10: X-ray structure of trans-[Cu(SCN)2(4-MeOPy)2]…………………………………...51 Figure 11: X-ray structure of trans-[Cu(SCN)2(4-AcPy)2]……………………………………..52 Figure 12: X-ray structures of new D4h monomers……………………………………………..54 Figure 13: X-ray structures of new C4v dimer complexes………………………………………55 Figure 14: X-ray structure trans-[Cu(SCN)2(4-NH2Py)2]………………………………………56 Figure 15: X-ray structures of new MeOH-containing complexes……………………………...58 4 Figure 16: X-ray structure of trans-Cu[(SCN)2(µ-OMe)2(2-NH2Py)2]…………………………59 Figure 17: X-ray structure of new network complexes…………………………………………60 Figure 18: Correlation of Cu…S bond length with pyridine substituent position……………….61 5 Acknowledgments This project, to say nothing of my entire undergraduate chemistry career, is deeply indebted to the guidance and diligent effort of Dr. Robert Pike, who has been a mentor and advisor to me through four years at William & Mary. I would like to thank Dr. Bebout, Dr. McNamara, and Dr. Grandis for serving as my thesis committee, with a special nod to Dr. Grandis for humoring my desire to drag my orchestra conductor into my chemistry major. Lastly, I wish to thank the William & Mary chemistry department for an incredible four years of support and opportunity. 6 Abstract: Twenty-one novel complexes of copper(II) thiocyanate pyridine were synthesized in methanol from the source material Cu(NO3)2•2.5H2O and a diverse series of substituted pyridines (XPy). These complexes typically formed according to the formula trans-[Cu(NCS)2(XPy)2], with square-planar geometries that exhibited thiocyanate bridging via long Cu–S bonds. The length of this long bridging bond appears to loosely correlate to the substitution position of the pyridine ligand used, and resulted in square planar molecules forming edge-sharing chain networks of Jahn- Teller distorted octahedra. Several other structural types were also observed, resulting in part from the tendency of some complexes to form methanol solvates. Several species displayed polymorphism with known complexes or each other. Methanol-solvate structures include square- pyramidal monomers and networks of the form trans-[Cu(NCS)2(XPy)2(MeOH)], as well as Jahn- Teller distorted octrahedral monomers of the form trans-[Cu(NCS)2(XPy)2(MeOH)2]. The ligand 2-NH2Py produced an unusual network of methoxy-bridge dimers, trans-[Cu(NCS)2(2- NH2Py)2(µ-OMe)2], that are further linked via bridging thiocyanate to form a sheet structure. Furthermore, a mixed-ligand solution of (2-BrPy) and (3-BrPy) formed the surprising complex trans-[Cu(NCS)2(2-BrPy)(3-BrPy)]. Bridging complexes, trans-[Cu(NCS)2(LL)], were also formed from the ligands LL = 4,4ʹ-bipyridyl (Bpy) and pyrazine (Pyz); in these cases, bridging by both the organic and thiocyanate ligands produced sheet networks. 7 Introduction: Copper is a transition metal with high natural abundance whose myriad distinct and useful qualities have been observed since antiquity. In modern chemistry, its status as an electron-rich and abundant group-11 metal has made it the subject an enormous variety of research and practical applications ranging from alloys to semiconductors to solar cells to catalysis. In its non-oxidized, pure metallic state, copper(0) is easily recognizable by its brown-gold luster. Metallic copper has been mined by human civilizations for millennia and valued for its color, malleability, resistance to corrosion, and electrical conductance. In 2015, world copper production exceeded 19 billion tons, with most metal going towards production of electronics, roofing and plumbing, and industrial machinery.1 In the world of coordination chemistry, copper is most commonly encountered in its +1 and +2 oxidation states. Copper(I) is the rarer of the two. It forms compounds that can be colorless or range in color from red to yellow, and its filled d10 count gives it a number of unique electronic and photophysical properties. However, it is normally less stable than copper(II). Copper(II), conversely, is the most common oxidation state of copper, and usually forms stable complexes that are normally green or blue in color. The tendency of copper(II) to behave predictably and form stable complexes, as well as the practical ease of obtaining and working with copper(II) source compounds, makes it an attractive object of study, and it is this oxidation state of copper that was the subject of investigation by this work. Electronic Orbitals and Crystal Field Splitting in Copper(II) The principal factor in describing any metal is the behavior of its valence electrons. Copper(II) has an electron configuration of [Ar]3d9. The fact that this configuration is only one 8 electron less than a full, 10-electron 3d subshell is very relevant to the bonding and coordinating behavior of copper(II), and makes it strongly favor low-spin geometries in complex with ligands- A ligand is simply any ion or molecule that binds to a metal, either by donating a lone pair of electrons (L-type) or a single electron (X-type) into the metal center, usually to reach a stable total of 18 (or sometimes 16) electrons.2 The arrangement of electrons around any metallic nucleus is well-described by electronic orbitals: volumetric spaces with quantized geometries that represent the probability distribution of an electron in three-dimensional space. The outermost occupied electron shell of copper, like all transition metals, is the d subshell, which consists of five distinct orbitals whose shapes and conventionally-defined orientations are displayed below (Figure 1). Each of these orbitals contains up to two electrons, for a total of 10 possible electrons in the d subshell. Copper(II), of course,
Details
-
File Typepdf
-
Upload Time-
-
Content LanguagesEnglish
-
Upload UserAnonymous/Not logged-in
-
File Pages69 Page
-
File Size-