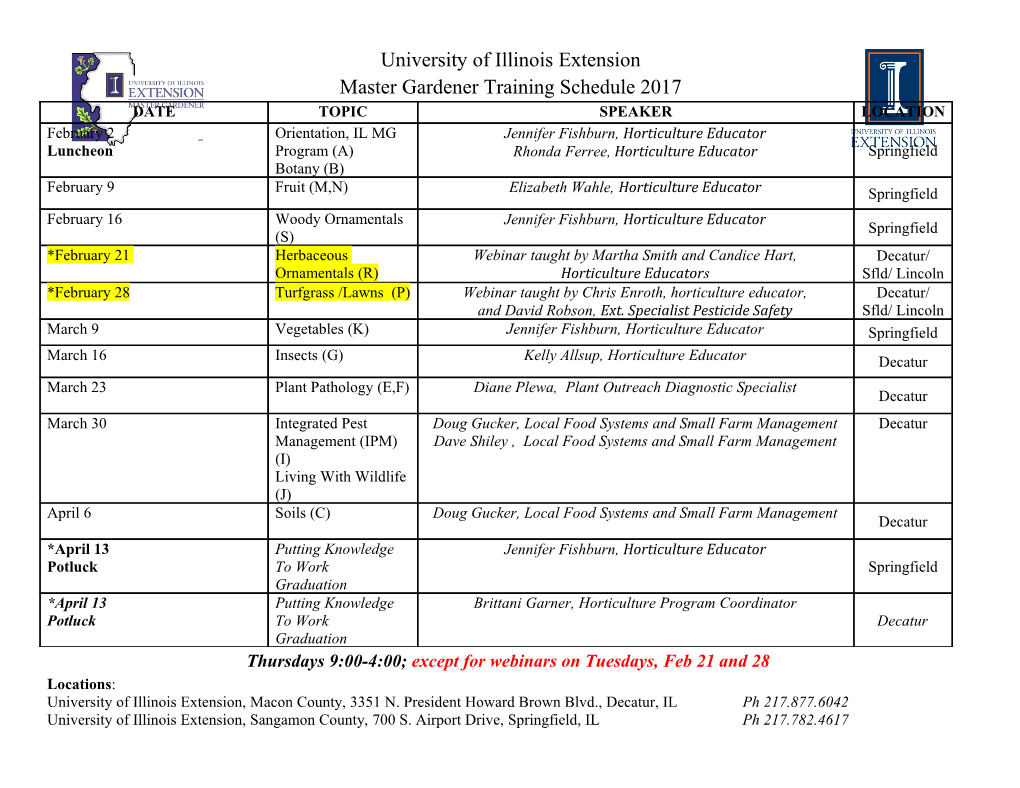
U-series constraints on intraplate magmatism Bernard Bourdon1 and Ken Sims2 1 Laboratoire de Géochimie et Cosmochimie, IPGP-CNRS UMR7579, 4, Place Jussieu, 75252 Paris cedex 05 France 2 Department of Geology and Geophysics , Woods Hole Oceanographic Institution, Woods Hole, MA 02543, USA 2 I. Introduction Intraplate magmatism represents approximately one tenth of the flux of magma to the Earth’s surface (Sleep 1990). This type of magmatism has received much attention from petrologists and geochemists as it generally exhibits a wide range of chemical compositions compared with the more uniform mid-ocean ridge basalts. Hence, it is rather paradoxical that our understanding of intraplate magmatism is rather poor. In this chapter, we shall attempt to review what insights have been gained from using U-series to constrain the sources and processes related to intraplate volcanism. An important feature of hotspot magmatism is that in many cases, the timing of hotspot activity seems to be decoupled from the motion of the lithospheric plate. This observation, which has been the basis for proposing the existence of mantle plumes, suggests that magmas erupted at hotspots should reveal something about the nature of the deeper mantle. Understanding the processes of hotspot magmatism should also tell us about the nature of convective motion that are responsible for hotspots. A major question about hotspots is the depth from which they originate. If they originate from the seismic discontinuity at 670 km, then, their composition tells us little about the lower mantle and the exchange between the lower and upper mantle must be restricted to a diffuse return flux. If, on the other hand, they originate from the core-mantle boundary, then they should provide some information about the D" and the global convective motion of the mantle. In the recent years, hybrid models with moving boudaries have been proposed and the boundary layer generating mantle plumes are not necessarily restricted to the cases mentioned above. There is considerable stake in better understanding the origin and nature of hotspot material. In this contribution, we shall not specifically challenge the link between mantle plumes and hotspots that has been a paradigm in the Earth Sciences (Wilson 1963; 3 Morgan,1971). We shall see however that several observations that are made with U-series in fact support this theory. In the following section, we first review some of the outstanding issues that need to be resolved to better understand intraplate magmatism. We then discuss some of the specific advances that were possible using U-series. Specific features related to magma differentiation are dealt with in the chapter by Condomines et al. (this volume). II. Difficulties in constraining hotspot melting processes : II.a Source composition and source heterogeneities It has long been known that the source of hotspots is different from the source of mid- ocean ridge magmatism (Gast 1968) both on the basis of trace element systematics (e.g; rare earth elements) and long-lived isotope systems (Rb-Sr, Sm-Nd, U-Pb). Both types of tracers indicate an enrichment of hotspot material relative to the depleted mantle sampled at mid- ocean ridges. What is less clear is whether this source material is also distinct in terms of its major elements (Langmuir and Hanson 1980). There are two main reasons why this debate is still ongoing: firstly, as hotspot magmas often represent smaller extents of melting (Gast, 1968 , the experimental constraints on the composition of these lavas are difficult to obtain because it has been more difficult to perform experiments with low extents of melting until recently (Baker et al., 1995). Secondly, the amount of volatiles (CO2 and H2O) is generally greater in hotspot lavas than in mid-ocean ridge basalts (MORB). These volatiles will significantly depress the solidus temperature at a given pressure (Olfasson and Eggler 1983; Falloon and Green 1989, see Figure 1). The melt productivities will also be affected and the composition of melts will be greatly different. As shown by the early work of Kushiro (1968), a greater pressure in CO2 will result in more undersaturated compositions while water will have the opposite effect. Recovering the initial source composition is difficult, such that a 4 full parameterization of all these effects is not a trivial task and often results in non-unique solutions. It has also been recognized that some minor phases, such as hydrous phases (amphibole, phlogopite), or accessory phases (rutile, apatite, etc.) are sometimes present in the source of hotspots (Class et al. 1997, 1998 ; Späth et al. 1996; Hoernle et al. 1993). These phases have a strong potential for fractionating the trace elements that can be used to trace both the nature of the source material and the melting process (LaTourrette et al. 1995; Sigmarsson et al. 1998). For trace element geochemists, this has been a major challenge to identify the presence of these phases and in some cases, invert the data to calculate the bulk partition coefficients and degree of melting (Minster et al. 1978; Sims et al. 1995). The presence of hydrous phases makes it more difficult to parameterize melting reactions and predict melt compositions for a given pressure and temperature because there are fewer experimental constraints (Wendlandt and Eggler, 1980; Olfasson and Eggler 1983). An added complexity stems from the generally large compositional range found in a single locality (e.g. Dupré et al. 1982). This is often interpreted as reflecting heterogeneities within the source of the hotspot (e.g.Lassiter and Hauri 1998). Up to five distinct components have been identified on a global basis (Zindler and Hart 1986). Some of the components can be clearly fingerprinted such as the N-MORB mantle source or the lithospheric mantle. It is far more difficult to establish what the composition of recycled components should look like (White and Hoffmann 1982; Staudigel et al. 1995; Hart 1989). One of the debates has been to decide whether a pyroxenitic component is present in the source of ocean island lavas in addition to a peridotitic component (Allègre and Turcotte 1985; Hirschmann et al. 1995; Sigmarsson et al. 1998). Melting of a pyroxenite should yield compositions and melting temperature (Figure 1) that are markedly different from a peridotite. Unfortunately, there are very few constraints on the major element composition of pyroxenite melts. Furthermore, the 5 modal abundances of minerals in pyroxenite differs greatly from peridotite which should also affect the relative elemental fractionation during melting. II.b Role of the lithosphere In most cases, the lithosphere acts as an upper boundary in the melting regime of hotspots except when they are located on the axis of a spreading center (Iceland, Galapagos). Thus, this cold lithospheric cap adds yet another complexity in understanding hotspot magmatism. Firstly, the depth of the base of the lithosphere is not always well known which means that the final pressure of melting is not always well constrained. Secondly, the lithosphere will act a cold boundary that will pertub the temperature field created by upwelling of hot material (Watson and MacKenzie 1991). It is considered that the lithosphere will only be able melt once the lithosphere has been heated by the impinging plume (Chase and Liu, 1991; Ribe 1988). This process should take a few 10-20 Ma considering the rate of heat diffusion in solids and the temperature-dependence of viscosity (Olson et al. 1988). Even if the lithosphere does not melt extensively, the interaction of plume melts with the lithosphere can potentially affect the trace element and isotope signatures of hotspot magmas (Chase and Liu 1991; Class et al. 1997, 1998). While we do have constraints on the composition of the lithosphere from peridotite nodules carried by hotspot magmas, the information they carry is not always relevant to understanding the origin and composition of hotspot magmas. For example, the pressure determined on the basis of fluid and melt inclusions can indicate an intermediate pressure of 10 kbar (Schiano et al. 1998). This would indicate that the nodule come from the middle of the lithosphere and not from its base where magmas are likely to interact. As the lithosphere has been stable over a timescale of up to several Ga, it may have had a complex history of depletion followed by metasomatism (Pearson et al. 1995; Hawkesworth et al. 1989). The 6 addition of minute amounts of metasomatic melt can wildly fractionate trace elements (Galer and O'Nions 1986; MacKenzie 1989) and ultimately result in long-lived isotope signatures (Sr, Nd and Pb) that are significantly different from the asthenospheric mantle (Hawkesworth et al. 1990). II.c Complexities in the melting region The presence of a lithosphere with a thickness up to 100 km above the plume head obscures the observations that could be made in terms of heat flow, gravity field or seismic structure. Establishing the temperature and flow fields beneath a hotspot thus becomes a difficult exercise. Several key parameters (Fig. 2) are poorly defined and mostly results from theoretical fluid dynamics model which underlines their large uncertainty. The temperature beneath hotspot region is generally estimated to be approximately 200±100°C with large uncertainties (Shilling 1991; Sleep 1990). The temperature anomalies will induce smaller densities in the plume and the flux of the density anomalies is called buoyancy flux as defined in (Sleep 1990): BQ==DDrra.. TQ . (1) where Q is the volume flux, r the density, T the temperature and a the coefficient of thermal expansion. Since the contribution of Sleep (1990) about buoyancy fluxes determined based on a fixed point approach, there has been several refinements for modelling more the buoyancy fluxes beneath hotspots (Ribe, 1996). It has been argued for example that a good estimate of buoyancy flux should take into account the excess buoyancy gained from melt depletion (Ribe and Christensen 1999).
Details
-
File Typepdf
-
Upload Time-
-
Content LanguagesEnglish
-
Upload UserAnonymous/Not logged-in
-
File Pages70 Page
-
File Size-