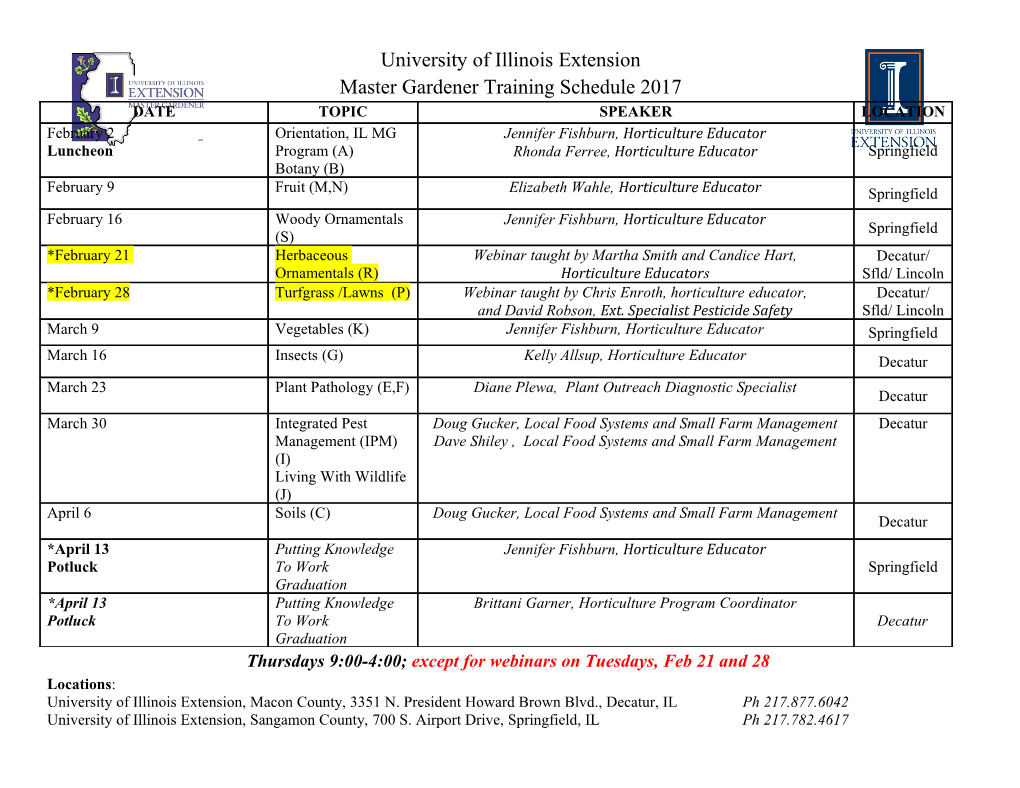
15 Projective Special Linear Group P SL(n; F ) Definition 15.1. The projective linear group and projective special linear group are the quotients of GL(n; F ) and SL(n; F ) by their centers: GL(n; F ) GL(n; F ) P GL(n; F ) = = Z(GL(n; F )) F £ SL(n; F ) SL(n; F ) P SL(n; F ) = = Z(SL(n; F )) f® 2 F £ j ®n = 1g These groups act on the n ¡ 1 dimensional projective space P n¡1(F ) (the set of one dimensional subspaces of F n) by the following lemma. Lemma 15.2. The only elements of GL(n; F ) which stabilize every element of P n¡1(F ) are the scalar multiples of the identity matrix. Proof. If A 2 GL(n; F ) stabilizes the spans F ei of the standard basis vectors n £ ei of F then Aei = ¸iei for some ¸i 2 F so A = diag(¸1; ¢ ¢ ¢ ; ¸n). We claim that ¸i = ¸j for all i; j since, otherwise, A will not stabilize F (ei + ej): A(ei + ej) = ¸iei + ¸jej 2 F (ei + ej) , ¸i = ¸j Thus A = ¸In. This implies that we get an induced action of P GL(n; F ) on P n¡1(F ) which is faithful in the sense that only the identity group element fixes every point in P n¡1(F ). We also get an induced action of P SL(n; F ) on P n¡1(F ). Identifying these groups with the corresponding symmetry groups on P n¡1(F ) we get: P SL(n; F ) · P GL(n; F ): In this section we want to prove that P SL(n; q) is a simple group for n ¸ 2 with two exceptions. Following Alperin we use the fact that it acts doubly transitively on projective space. Definition 15.3. The action of a group G on a set X is called doubly tran- sitive if G acts transitively on the set of all ordered pairs of distinct elements of X. In other words, given any x1; x2; y1; y2 2 X so that x1 6= x2 and y1 6= y2 there is a g 2 G so that gxi = yi for i = 1; 2. Lemma 15.4. If G acts doubly transitively on a set X then the stabilizer Hx of any x 2 X is a maximal subgroup of G. Proof. Suppose that Hx is not maximal. Then there is a subgroup K so that G > K > Hx. Thus there is a g 2 G, g2 = K and k 2 K, k2 = Hx. Since g; k are not in Hx they do not stabilize x. Thus gx; kx are not equal to x. Since G acts doubly transitively on X, there is an h 2 G so that hx = x and ¡1 h(gx) = kx. But then h; k hg 2 Hx < K so ¡1 g 2 h kHx ⊆ K which is a contradiction. 1 Lemma 15.5. If n ¸ 2, the action of SL(n; F ) and thus of P SL(n; F ) on projective space is doubly transitive. n¡1 n Proof. Suppose that L1;L2 are distinct elements of P (F )[Li ⊆ F ]. Then any nonzero vectors vi 2 Li will be linearly independent so can be extended n to a basis for F . Thus there is an A 2 GL(n; F ) so that Aei = vi for i = 1; 2. Let ¸ = det(A). Then B = A diag(¸¡1; 1; ¢ ¢ ¢ ; 1) 2 SL(n; F ) ¡1 sends e1 to ¸ v1 and e2 to v2. So BF ei = Li for i = 1; 2. Lemma 15.6. Every Xij(®) 2 SL(n; F ) is conjugate to an elementary ma- trix of the form X12(¯). Proof. Let σ 2 Sn be a permutation of f1; ¢ ¢ ¢ ; ng so that σ(1) = i; σ(2) = j. Let ² be the sign of σ. Then there is an s 2 SL(n; F ) so that s(e1) = ²ei and s(ek) = eσ(k) for all 2 · k · n. Then sX12(²®) = s(e1; e2 + ²®e1; e3; ¢ ¢ ¢ ; en) = (²ei; ej + ®ei; eσ(3); ¢ ¢ ¢ ; eσ(n)) = Xij(®)(²ei; ej; eσ(3); ¢ ¢ ¢ ; eσ(n)) = Xij(®)s: ¡1 Consequently, s Xij(®)s = X12(²®). Theorem 15.7. For n ¸ 2, P SL(n; F ) is simple with the exception of P SL(2; 2) and P SL(2; 3). Proof. Let P be the stabilizer of F e1 in SL(n; F ). Then P has the following properties: 1. P is a maximal subgroup of SL(n; F ) by Lemmas 15.4 and 15.5. ¡1 2. For any s 2 SL(n; F ), sP s is the stabilizer of s(F e1). 3. The elements of P can be written uniquely in the form: µ ¶ a v 0 B £ n¡1 1 where a 2 F , v 2 F and B 2 GL(n ¡ 1;F ) with det(B) = a . To show that P SL(n; F ) is simple it suffices to show that any proper normal subgroup N of SL(n; F ) is contained in its center. Case 1. Suppose first that N · P . Then N = sNs¡1 · sP s¡1 for all s 2 SL(n; F ). Consequently, N stabilizes every one dimensional subspace n¡1 F v 2 P (F ) and thus consists of scalar multiples of In by Lemma 15.2. Case 2. Now suppose that N is not contained in P . Then PN = SL(n; F ) by maximality of P . So for any K E P , we have KN E SL(n; F ) (since both P and N normalize KN). Let K be the group of all matrices of the form: µ ¶ 1 w 0 In¡1 2 This is a normal subgroup of P since: µ ¶ µ ¶ µ ¶ µ ¶ µ ¶ a v 1 w a v + aw 1 awB¡1 a v = = 0 B 0 In¡1 0 B 0 In¡1 0 B K is also abelian, being isomorphic to the additive group F n¡1. Since KN E SL(n; F ) and every generator Xij(¸) of SL(n; F ) is conjugate to an element of K by Lemma 15.6, we must have KN = SL(n; F ). But then SL(n; F ) K »= N K \ N is abelian (since K »= F n¡1 is abelian). By Theorem 14.8 this is impossible except in the cases of SL(2; 2) and SL(2; 3). Other matrices Permutation matrices were used in the proof of Lemma 15.6. If σ 2 Sn then the corresponding matrix Á(σ) 2 GL(n; F ) is given by Á(σ)(ei) = eσ(i). In words this is the matrix obtained from the identity matrix In by permuting its rows by σ. Thus, e.g., 0 1 0 0 1 Á(123) = @1 0 0A 0 1 0 This gives a homomorphism Á : Sn ! GL(n; F ) whose image Alperin calls W because it is the Weyl group in this case. The (standard) Borel subgroup of GL(n; F ) is the group of invertible upper triangular matrices: 80 19 < ¤ ¤ ¤ = B = @0 ¤ ¤A : ; 0 0 ¤ Note that this includes all diagonal matrices and all Xij(¸) where i < j. Theorem 15.8. GL(n; F ) = BW B. Proof. Take any A 2 GL(n; F ). Let anj be the first nonzero entry in the bottom row. Then by right multiplication by some b 2 B we can change this entry to 1 and clean (make into 0) the rest of the bottom row. By left multiplication by some b0 2 B we can clean the rest of the j-th column. Now suppose that the first nonzero entry in the n ¡ 1st row of b0Ab is the (n ¡ 1; k) entry. Then we can make it 1 and clean the rest of that row and column. Continuing in this way we find b1; b2 2 B s.t. b1Ab2 2 W so ¡1 ¡1 A 2 b1 W b2 ⊆ BW B. 3 This theorem says that GL(n; F ) is a union of the double cosets BwB. The following lemma implies that these double cosets are disjoint. Lemma 15.9. Suppose that w1; w2 2 W and b 2 B so that w1bw2 2 B. ¡1 Then w2 = w1 . Proof. For each t 2 F let b(t) be the matrix obtained from b by multiplying the off diagonal entries by t. Then w1b(t)w2 2 B for all t 2 F . In particular this is true for t = 0. But b(0) is a diagonal matrix so w1b(0)w2 2 B implies w1w2 = In. Theorem 15.10 (Bruhat decomposition). The double cosets BwB are disjoint. Consequently, GL(n; F ) can be expressed as a disjoint union: a GL(n; F ) = BwB w2W 0 Proof. Suppose that BwB intersects Bw B. Then there are elements bi of B 0 so that b1wb2 = b3w b4. But this implies ¡1 ¡1 0 ¡1 w b1 w = b4b2 2 B so w = w0 by Lemma 15.9. 4.
Details
-
File Typepdf
-
Upload Time-
-
Content LanguagesEnglish
-
Upload UserAnonymous/Not logged-in
-
File Pages4 Page
-
File Size-