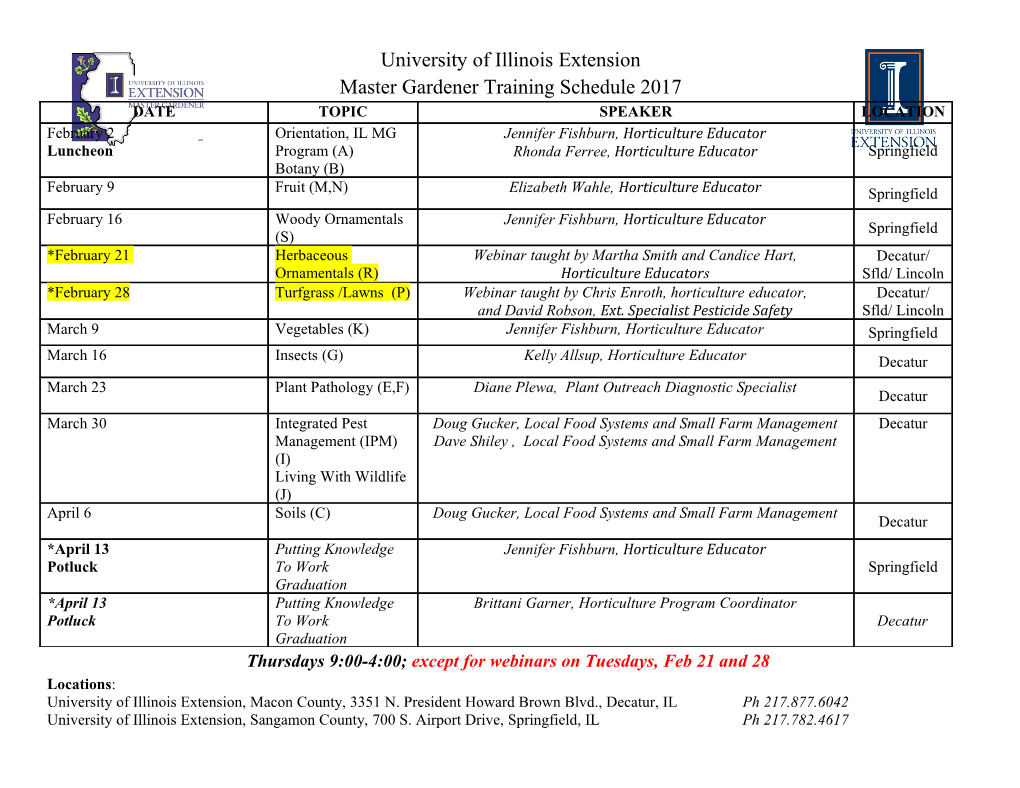
Crystallisation and Structural Studies on Omega Transaminase Enzymes Submitted by Aaron Charles Westlake to the University of Exeter for the degree of Masters by Research in Bioscience March 2012 This report is available for Library use on the understanding that it is copyright material and that no quotation from the report may be published without proper acknowledgement. I certify that all material in this report which is not my own work has been identified and that no material has previously been submitted and approved for the award of a degree by this or any other University. Aaron Westlake Supervisor: Professor Jennifer Littlechild Abstract Omega transaminase enzymes are proving to be of vital importance in the production of chiral amines for drug manufacturing due to their high stereoselectivity and regioselectivity reactions. Omega transaminases also possess another key feature that can make them more desirable than other transaminase enzymes in that they are able to utilise substrates lacking a carboxyl group. This project has purified several recombinant omega transaminases from the bacteria; Streptomyces avermitilis, Deinococcus geothermalis, Pseudomonas putida and Pseudomonas aeruginosa. Attempts have been made to crystallise the proteins in order to determine their structures. D. geothermalis omega transaminase failed to purify due to lack of binding to Nickel columns despite having a histidine tag. P. putida omega transaminase failed to produce any crystals in the crystal screens available. P. aeruginosa omega transaminase crystals, whose holoenzyme structure has already been solved, were soaked in pyruvate and also produced crystals from co-crystallisation experiments with pyruvate. These crystals however did not show sufficient electron density in the active site to determine if the substrate had bound. They did however diffract to 2.0 Å and 1.8 Å resolution respectively. Co-crystallisation experiments with P. aeruginosa omega transaminase and the inhibitor gabaculine were successful however to date they have failed to produce X-ray diffraction data that could be processed despite diffracting to high resolution. The S. avermitilis omega transaminase produced crystals that were shown to be disordered when they underwent X-ray diffraction. Differential scanning fluorometry was carried out to identify the optimal buffer for protein stability. This identified the current Tris pH 7.5 buffer to lead to a low thermodynamic stability of the protein. Several new buffers including MES at pH 6 were identified as being the most likely candidates for stabilising the protein and producing ordered crystals. Data collected from a Chromobacterium violaceum omega transaminase with a F89 to A89 mutation was successfully processed and refined. The resultant structure showed an increase in flexibility in the substrate carboxyl binding residue, R416, and an increase in space available for substrate binding. HPLC assays showed alpha-methylbenzylamine activity remained. An amino acid oxidase/horseradish peroxidase linked assay showed there to be a small increase in activity towards β-alanine due to this mutation. Further mutations will be necessary to enable this enzyme to utilise β-alanine at a more practical rate of conversion. 2 Abbreviations 4-AAP 4-Aminoantipyrine AcornAT Acetylornithine aminotransferase AlaAT Alanine aminotransferase AP Acetophenone APS Ammonium persulfate AT Aminotransferase AspAT Aspartate aminotransferase BcaaAT Branched chain amino acid aminotransferase Da Daltons DaaAT D-amino acid aminotransferase D-alaAT D-alanine aminotransferase DapaAT 7,8-Diaminopelargonic acid aminotransferase dd Double distilled EPPS 4-(2-Hydroxyethyl)-1-piperazinepropanesulfonic acid GabaAT γ-Aminobutyric acid aminotransferase GF Gel filtration HEPES 4-(2-Hydroxyethyl)piperazine-1-ethanesulfonic acid HisPAT Histidinol-phosphate aminotransferase His-tag Poly histidine tag IPTG Isopropyl β-D-galactopyranoside MBA α-methylbenzylamine MES 4-Morpholineethanesulfonic acid MOPS 4-Morpholinepropanesulfonic acid mPMS 1-Methoxy-5-Methylphenazinium Methyl Sulfate MTT 3-(4,5-Dimethylthiazol-2-yl)-2,5-diphenyltetrazolium bromide NAD Nicotinamide adenine dinucleotide OrnAT Ornithine aminotransferase PAGE Polyacrylamide gel electrophoresis PDB Protein Databank PheAT Phenylalanine aminotransferase PIPES 1,4-Piperazinediethanesulfonic acid PLP Pyridoxal-5’-phosphate PMP Pyridoxamine phosphate PSerAT Phosphoserine aminotransferase SDS Sodium dodecyl sulfate SerAT Serine aminotransferase 3 TBHBA 2, 4, 6-Tribromo-3-hydroxybenzoic acid TEMED N,N,N,N-tetramethylethylene diamide Tris Tris[hydroxymethyl]aminomethane TyrAT Tyrosine aminotransferase ω-AaAT ω-amino acid aminotransferase 4 List of Contents Chapter 1 - Introduction 1.1. Applications of Biocatalysis...…………………………………………………….7 1.2. Transaminase Enzymes...………………………………………………………....8 1.3. Transaminase Mechanism………………………………………………………...9 1.4. Transaminase Classification……………………………………………………..11 1.5. Omega Transaminase Specificity………………………………………………..12 1.6. Omega Transaminase Structure………………………………………………….13 1.7. Inhibition of Omega Transaminase……………………………………………...15 1.8. Differential Scanning Fluorometry Theory……………………………………...17 1.9. Previous Work Relevant To This Project 1.9.1. Structural Studies on Native Omega Transaminase…………………...18 1.9.2. Site Directed Mutagenesis of Omega Transaminase…………………..19 1.10. Aims of Project.………………………………………………………………...20 Chapter 2 - Materials and Methods 2.1. Enzyme Purification 2.1.1. Buffers....……………………………………………………………....22 2.1.2. Bacteria Cultures and Storage…………………………………………23 2.1.3. Lysing Cell Cultures…………………………………………………...23 2.1.4. SDS-PAGE gels…………………………………………………....….24 2.1.5. Purification of enzyme………………………………………………...25 2.1.6. Nickel Chromatography……………………………………………….25 2.1.7. Hydrophobic interaction Chromatography…………………………….25 2.1.8. Ion exchange Chromatography………………………………………..25 2.1.9. Gel Filtration Chromatography………………………………………..26 2.2. Crystallisation Trials 2.2.1. Protein Concentration Determination………………………………….26 2.2.2. Crystal Screening..…………………………………………………….27 2.2.3. Crystal Soaks..…………………………………………………………27 2.2.4. Co-crystallisation..………………………………………....………….28 2.2.5. Crystal Optimisation..……………………………………....…………28 2.2.6. Freezing Crystals..……………………………………………………..28 5 2.3. Assays 2.3.1. HPLC Assay.....………………………………………………………..29 2.3.2. Tetrazolium Salt Based High-Throughput Assay……………………..30 2.3.3. Amino Acid Oxidase/Horseradish Peroxidase Linked Assay…………30 2.3.4. Differential Scanning Fluorometry..…………………………………..31 2.4. Bioinformatics 2.4.1. Multiple Sequence Alignment…………………………………………32 2.4.2. Homology Modelling..………………………………………………...32 2.4.3. X-ray Data Collection..………………………………………………..32 2.4.4. Structural Refinement…………………………..……………………..32 Chapter 3 - Results 3.1. Protein Purification 3.1.1. Successful Purification Experiments…………………………………..33 3.1.2. Attempts at the Purification of D. geothermalis omega transaminase...37 3.2. Protein Crystallisation and Structural Determination 3.2.1. Crystallisation Trials…………………………………………………..40 3.2.2. Crystal Soaks…………………………………………………………..43 3.2.3. Co-crystallisation Trials……………………………………………….43 3.3. Structural Analysis 3.3.1. Multiple Sequence Alignment…………………………………………49 3.3.2. Homology Modelling Analysis………………………………………..51 3.3.3. Structural Refinement of Mutant C. violaceum omega transaminase…53 3.4. Activity Assays………………………………………………………………….56 3.5. Differential Scanning Fluorometry……………………………………………...58 Chapter 4 – Discussion……………………………………………………………...62 Chapter 5 - Conclusion 5.1. Summary of Work……………………………………………………………….65 5.2. Future Work.…………………………………………………………………….66 5.3. Acknowledgements.……………………………………………………………..66 References…………………………………………………………………………...67 6 Chapter 1 - Introduction 1.1. Applications of Biocatalysis Recently enzymes are being utilized as biocatalysts in a myriad of industrial processes for their high specificity, consistent results and, unlike tradition manufacturing materials, usually involving high temperatures, high pressures, toxic transition metals or a combination of the three, are reusable and can be highly stereoselective [1]. Examples of biocatalytic processes include the breakdown of amides by penicillin acylase, hydrolysis of esters to acids by esterases and lipases, the formation of malic acid by condensation by fumarase, conversion of glucose to fructose by glucose isomerase and the removal of a halogen by dehalogenases [2]. Many semi-synthetic antibiotics are now being made by utilising biocatalysis instead of chemical conversions based on stoichiometry. α-amino acid ester hydrolase, α-acylamino-β- lactam acylhydrolase and ampicillin acylase are all enzymes that have been reported capable of producing semi-synthetic cephalosporins from 7-aminocephem or 6- aminopenam with α-amino acid esters [3]. The immobilized enzyme penicillin acylase is being used in the manufacturing process of β-lactam antibiotics. Condensation of 6- aminopenicillanic acid with D-(−)-4-hydroxyphenylglycine or D-(−)-phenylglycine by this enzyme will make amoxicillin or ampicillin respectively. Also cefadroxil and cephalexin can be made from 7-aminodesacetoxycephalosporanic acid in the same manner [4]. Whole cells can be used in complex reaction pathways that require regeneration of co-factors for the enzymes as it is far easier and cheaper to allow a living cell to do all the work in ensuring the enzyme remains active [1]. Due to their high specificity, there are few by-products of enzyme based reactions which
Details
-
File Typepdf
-
Upload Time-
-
Content LanguagesEnglish
-
Upload UserAnonymous/Not logged-in
-
File Pages71 Page
-
File Size-