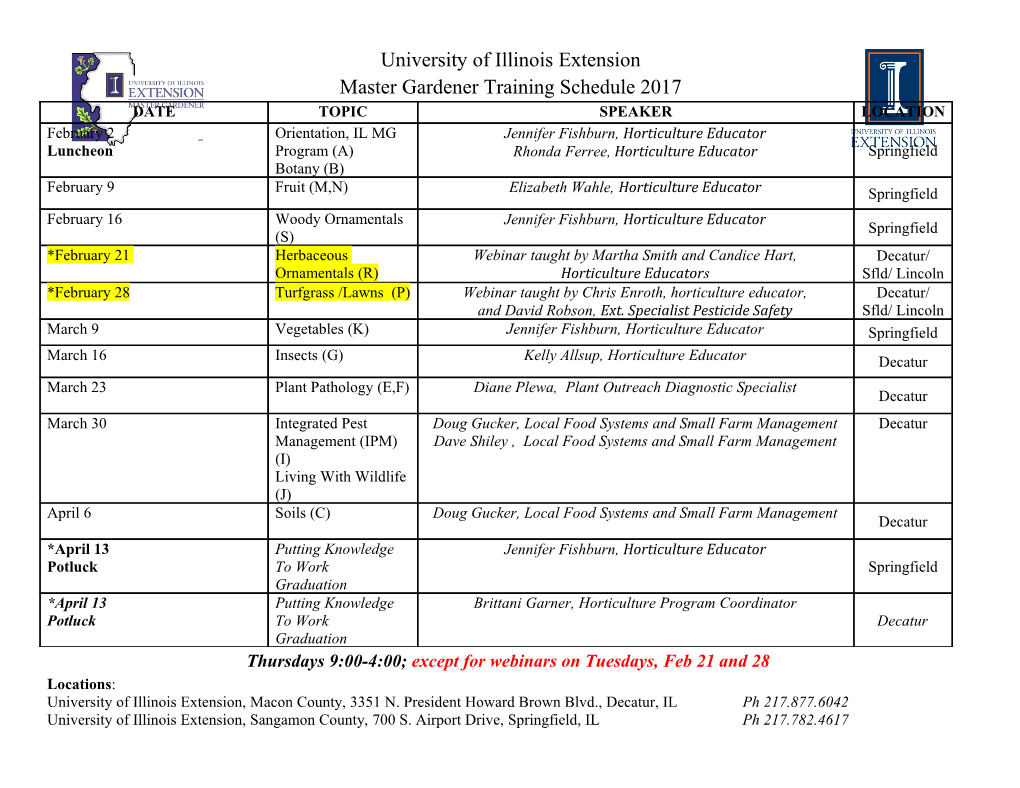
Comparison of the Current Field in Fram Strait derived from ADCP Measurements and Mooring Data Master Thesis Postgraduate Programme Environmental Physics Axel Behrendt 30.09.2008 Supervisor: Prof. Dr. Ursula Schauer Second Referee: Prof. Dr. Monika Rhein Contents i Contents 1 Introduction 1 1.1 Arctic Ocean Warming . 3 1.2 Mass Budget and Volume Fluxes . 4 2 Motivation and Scientific Goals 7 3 The Arctic Ocean 9 3.1 Geographical Setting . 9 3.2 Circulation and Hydrography . 11 4 Techniques and Methods 16 4.1 Moorings and Instruments . 16 4.2 ADCP . 18 4.3 Tidal Analysis . 19 4.3.1 Harmonic Analysis with T_TIDE ...................... 21 4.3.2 The AOTIM-5 Model . 23 5 Results and Discussion 24 5.1 Tidal Analysis of the Mooring Data . 24 5.2 Comparison with Model Results . 30 5.3 LADCP Data and Tidal Correction . 32 5.4 Volume Transports . 34 5.4.1 Transports from LADCP Data . 34 5.4.2 Combined Transports from Moorings and LADCP Data . 36 5.5 Uncertainties . 38 5.6 Summary . 40 6 Conclusions and Outlook 41 References 46 Appendix 49 Acknowledgements Acknowledgements I am grateful to my supervisor Dr. Ursula Schauer for the opportunity to work in the exciting field of physical oceanography and for the great support of my work. I wish to thank her and also Dr. Eberhard Fahrbach for making available the opportunity of participating in the Polarstern cruise ARK XXIII-2. This was a most fascinating experience! On this occasion, I thank the whole oceanography group and the crew on board Polarstern for the great time we had. I am especially thankful to Agnieszka Beszczynska-Möller and Benjamin Rabe for a lot of useful hints, papers, MatLab tools and their presence, whenever I had problems with my LADCP data. I want to thank my second referee Prof. Monika Rhein and Dr. Christian Mertens from the University Bremen for the opportunity to speak in their seminar. My thanks also to Dr. Waldemar Walczowski from the Institute of Oceanology in Sopot, who kindly provided some LADCP data for this study. Many thanks to the whole team of lecturers and the administration of the PEP program, especially to Anja Gatzka for her patience with sleepy students and all the help. Last but not least, some special words of gratitude to my parents, who consistently supported me whenever there were hard times to survive... Thank you !!! Axel Behrendt, Monday, 29. September 2008 Photo by Henri Robert (ARK XXIII-2, 2008) Acronyms List of Acronyms ABW Arctic Bottom Water ADCP Acoustic Doppler Current Profiler AO Arctic Ocean AOTIM Arctic Ocean Tidal Inverse Model ASW Arctic Surface Water AW Atlantic Water AWI Alfred Wegener Institute for Polar and Marine Research BG Beaufort Gyre CTD Conductivity, Temperature, Depth EGC East Greenland Current GSDW Greenland Sea Deep Water IBCAO International Bathymetric Chart of the Arctic Ocean IHO International Hydrographic Organization IPCC Intergovernmental Panel on Climate Change LADCP Lowered Acoustic Doppler Current Profiler NAC Norwegian Atlantic Current NADW North Atlantic Deep Water NAO North Atlantic Oscillation NASC Norwegian Atlantic Slope Current NSIDC National Snow and Ice Data Center RMS Root Mean Square SSH Sea Surface Height TD Transpolar Drift THC Thermohaline Circulation WBC Western Boundary Current WSC West Spitsbergen Current Chapter 1: Introduction 1 1 Introduction After the release of the fourth IPCC Assessment Report, the Earth’s climate system attracted more interest. Not only the considerable rise in surface temperatures during the recent century but also sea level changes and the amount of globally melting ice masses are immense. Today, numerous international climate research programs are dedicated to get a better understanding of our climate system and to improve the knowledge about the human influence on climate. The Earth’s climate system is characterized by complex interactions between atmosphere, oceans, cryosphere and land surfaces including the marine and terrestrial biosphere. The oceans are a very large heat reservoir and they represent the long-term memory of our climate system. Moreover, they transport vast amounts of energy and contribute approximately as much as the atmosphere to the climatic conditions in our environment. Particularly the polar regions are increasingly recognized as being regions with considerable potential to affect global climate. On the other hand, polar regions are subject to multiple stressors and extremely vulnerable to climate change. Contrary to the Antarctic, large areas of the Arctic are covered with water and therefore receive a high amount of heat. Besides the Antarctic Peninsula, Arctic regions have been the most rapidly warming areas of the globe over the past several decades. The Arctic comprises some of the most sensitive elements of the global environment which are considered to respond rapidly to climate change. The observed melting of ice masses and the reduction of snow cover reduces the high albedo, which leads to reduced reflection of sunlight and thus to an accelerated warming effect (ice-albedo feedback). In addition, a reduced sea ice coverage may have a stronger effect on climate than mid-latitude sea surface temperature changes [Talley, 2002]. Thawing of Arctic permafrost can release enormous amounts of stored greenhouse gases, which in turn has consequences for the global greenhouse effect. Furthermore, the northern polar region is a huge habitat which includes both terrestrial and marine ecosystems and it sustains a human population of approximately 4 million residents in a harsh environment. Therefore, a rapid climate change will also affect the socio-economic system in this region. It is unequivocal that the Arctic undergoes considerable climatic changes and communities and ecosystems are already being affected. Today, there is distinct evidence of strong climatic changes. During the recent decades, the surface air temperatures in this region have warmed at approximately twice the global rate. Since a temperature minimum in the 1960s and 1970s, the averaged surface air temperatures north of 60◦N have increased by 1-2◦C[Anisimov et al., 2007]. The reduction of sea ice, glaciers, river and lake ice and a warming of permafrost throughout the Arctic is consistent with the recent increase of surface temperatures in this region. Arctic sea ice decreased drastically during the recent decades. Today it covers an area of 15 × 106 km2 at maximum extent, reducing to only 7 × 106 km2 in summer [Lemke et al., 2007]. In September 2007, Arctic sea ice retreated to the lowest level since satellite measurements began in 1979. The average sea extent for this month was 4.28 × 106 km2 [NSIDC, 2008]. Furthermore, the discharge of Eurasian rivers draining into the Arctic Ocean (AO) shows a Chapter 1: Introduction 2 significant positive trend [Peterson et al., 2002]. The AO includes areas of deep convection which represent an important driving mechanism for the thermohaline circulation (THC). The intensity of this large scale ocean overturning is crucially regulated by freshwater exported from the Arctic Ocean. The THC is an important part of the Atlantic heat conveyor, and a slow down of the circulation might have serious consequences for the climate in Europe [Hansen et al., 2004; Quadfasel, 2005; Rahmstorf, 2006]. The temperatures in the marine Arctic during the 20th century are marked by strong multi- decadal variations, and in the recent years several authors have reported on a warming of the Atlantic Water layer. Numerous modern synoptic hydrographic surveys have been made in this region and today there is evidence that the Arctic Ocean is in transition towards a warmer state [Polyakov et al., 2005]. The Gulf Stream and its northern extension, the North Atlantic Current, transport large amounts of energy and contribute to the moderate climate in Europe. The northernmost extensions of the North Atlantic Current transport Atlantic Water through Fram Strait into the North Polar region and provide the warmest water masses that enter the Arctic Ocean. The assessment of the impact of these water masses on the Arctic heat budget is one of the major tasks in the observational oceanography section of the Alfred Wegener Institute (AWI). In order to determine both the amount of heat transported northwards and the southward outflow of fresh and shallow surface waters and saline deep waters through Fram Strait, it is essential to know the transported water masses as precisely as possible. For these purposes, an intensive mooring program was established in Fram Strait from 1997 on. The AWI deployed an array of 12-16 moorings at 78.8◦N, to quantify the volume fluxes through the Fram Strait. The moorings are equipped with mechanical and acoustic current meters, measuring the ve- locity of the ocean currents, and additional sensors to determine salinity and temperature of the sea water. This measuring system, maintained in close cooperation with the Norwegian Polar Institute, covers Fram Strait from the eastern to the western shelf edge and is the only way to perform long-term observations throughout the year. Every year, during the recov- ery/deployment phase, additional temperature, salinity and velocity measurements have been made along the mooring section in Fram Strait. Fig. 1.1: Mooring array (white circles) in Fram Strait at 78.8◦N. Chapter 1: Introduction 3 1.1 Arctic Ocean Warming The inflow of warm Atlantic Water through Fram Strait has been known since Nansen (1902). Although its large contribution to the heat budget of the Arctic Ocean is known, it is not yet fully understood. The Arctic Ocean affects the global climate through (1) the surface heat balance, which is coupled to the stratification of water masses, and (2) the outflow from the Arctic Ocean into the major convective regions, which affects the THC [Aagaard and Carmack, 1994]. Perturbations in the upper ocean can lead to a heat release from warm intermediate Atlantic waters to the atmosphere.
Details
-
File Typepdf
-
Upload Time-
-
Content LanguagesEnglish
-
Upload UserAnonymous/Not logged-in
-
File Pages54 Page
-
File Size-