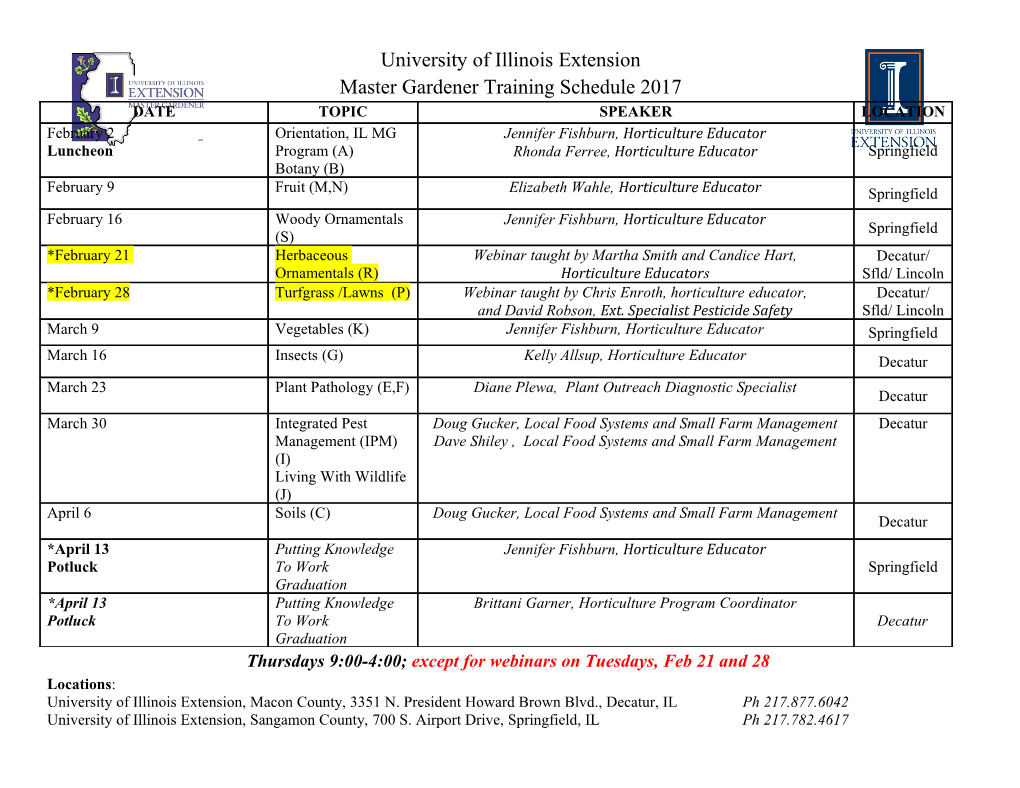
The mechanics of hearing Jonathan Ashmore 1 1 Introduction When we think of the ears, we think of the flaps that stick out from either side of our head. However these are only part of a complex physiological apparatus that enables us to hear all sorts of sounds and, more importantly, to respond appropriately. The most visible part of the ear is the outer ear, also sometimes called the external ear (Figure 1). The rest of the hearing organ, comprising the middle ear and the inner ear, is buried within the temporal bone on either side of the skull. The temporal bone can be felt just behind the outer ear. The inaccessibility of the structural components of hearing and, as we shall see, the small size of the important structures, makes the study of hearing particularly challenging. Figure 1 A cross section of the outer middle inner human ear. ear ear ear pinna malleus incus vestibular nerve cochlear nerve concha cochlea stapes oval external window Eustachian tube auditory eardrum canal round (tympanic window (meatus) membrane) We can hear because a precise sequence of events takes place in the ear. We shall explore these events in this and succeeding chapters. In this chapter, we shall trace the sequence of events from initial receipt of the signal to the stimulation of the cochlea. We use the word ‘ear’ as the collective description of all the structures and not just the outer ear. Normally when we hear a sound it is as a result of a physical disturbance transmitted through air, through the external auditory canal, through the middle ear and into the inner ear structures where it may be detected, if it is intense enough and of the right frequency. If you tap the temporal bone with your finger you can hear the percussive sound transmitted directly to the inner ear. In this case sound is said to be transmitted by bone conduction. This simple test is one of those used to identify whether hearing losses come about because sound is not being transmitted properly through the external and middle ear to the hearing organ of the inner ear or whether it is the inner ear itself that is working incorrectly. 4 SIGNALS AND PERCEPTION: THE FUNDAMENTALS OF HUMAN SENSATION 2 The sensitivity of the ear It is usually most convenient to describe the sensitivity of the ear in terms of a threshold. This threshold is the amplitude of a sound pressure wave that can just be heard when presented to a listener. To determine such a threshold it is important that the sound is delivered in completely quiet surroundings as any background sound can interfere with the measurement. Experiments have shown that the minimum amplitude of a sound wave that can be detected under optimal conditions for a normally-hearing individual is 20 µPa (micropascals). For comparison, atmospheric pressure is approximately 100 kPa, and hence a disturbance 2 × 10−10 times atmospheric pressure is detectable by the ear. An equivalent comparison is to consider the situation where you blow up a normal party balloon and then start pushing the tip of a pencil into the side. When the pencil indents the side by 1 mm, the pressure inside will have increased by about 20 µPa. Our ears, like microphones, are sensitive to sound pressure. A sound stimulus can be determined by physical measurements of the sound wave at the entrance to the external auditory canal using calibrated probe microphones. Sound is measured in units of decibels (dB SPL) where SPL stands for sound pressure level, and is defined as follows using a logarithmic scale: sound stimulus (in dB SPL) = 20 log10 (P/Pref) µ where Pref =20 Pa. This particular definition means that each increment of the sound wave amplitude by a factor of 10 will increment the sound level by 20 on the decibel scale. The definition is a consequence of sound energy being proportional to the square of the sound wave amplitude. The logarithmic scale is useful because of the very wide range of human hearing, where the amplitude of the sound varies by more than a million times, from the very softest that can be heard to the loudest, which can begin to produce damage. The energy per unit area (referred to as intensity) that passes into the ear from a sound wave with amplitude P is proportional to P2 and has units of W m−2. In air the threshold of hearing corresponds to 10−12 Wm−2. The threshold sound stimulus depends on the frequency. Figure 2 shows the auditory threshold curve (or audiogram) for a normal subject. The threshold rises at both lower and higher frequencies. Below about 20Hz, the sound has to be so intense that it is possible to say that there is no hearing below this frequency. Depending on the history and age of the individual, the auditory threshold also rises at higher frequencies. The range over which we hear is, optimally, 20Hz to 20kHz, but can be very different from individual to individual. In particular, the upper limit of hearing is severely reduced in age-related deafness. The manner in which the measured threshold curve differs from the curve obtained from a population of normally- hearing individuals is thus a measure of auditory performance. 3 Components of the ear: overall considerations Figure 3 shows the sequence of sound processing components in the ear. As a functional entity, these components constitute the peripheral auditory system. The function of the outer and middle ear is to transform sound from a wave travelling in air to one travelling in the fluids of the inner ear. This idea was first developed by the German scientist Hermann von Helmholtz in 1877 (Figure 4). ASHMORE THE MECHANICS OF HEARING 5 Figure 2 Auditory threshold 140 curve for a healthy young threshold of pain gun shots normally-hearing subject. With 120 ‘high risk’ rock concerts age, the threshold rises at the threshold higher frequencies. The auditory 100 road drill threshold curve for a cat is shown for comparison. 80 conversational 60 speech hearing 40 thresholds sound pressure / dB SPL 20 inaudible cat 0 human –20 10 100 1000 10 000 100 000 frequency / Hz Figure 3 Block diagram of the behaviour mammalian ear and its components, and its relation to the middle ear muscles central nervous system (CNS). efferent system outer ear middle ear inner ear CNS Figure 4 Hermann von Helmholtz (1821–1894). Helmholtz trained as a medical doctor before ultimately taking up the Chair of Physics in Berlin. He made many substantial contributions to physiology, and his book On the Sensation of Tone contains the first theoretical analysis of the process of hearing. 6 SIGNALS AND PERCEPTION: THE FUNDAMENTALS OF HUMAN SENSATION The effectiveness of the transmission in each structure of the ear is under central nervous system control. The outer ear can be oriented towards (or away from) a sound by movement of the head, and thus the intensity of sound entering each ear can be controlled. The middle ear, responsible for transmitting sound to the inner ear, contains two muscles that control the sound passing through. The inner ear itself is the target of a neural pathway (the efferent system) that can control the sensitivity of the sensorineural cells. Together these three subsystems determine the codes sent along the auditory nerve to the first relay nuclei of the auditory pathway. The descending control from the higher central nervous system therefore acts both to limit and, if necessary, to enhance the sound stimulus reaching the sensory structures of the inner ear and shows the importance of maintaining the correct level of stimulus. 4 The outer ear The outer ear includes the visible flap (pinna), the funnel-like inner portion (concha) and the external auditory canal (meatus) (Figure 1). The shape of our outer ears (and those of many mammals) is approximately that of a tapered tube with the larger end open to the outside of the head. The consequence of this shape is that sound is less effectively transmitted at frequencies below a critical frequency that depends on the length and cross-sectional areas of the meatus and concha. For the human ear this frequency is near 1–2kHz. In addition, there is sometimes a slight sympathetic vibration in the canal itself near 5–7 kHz, when the canal behaves like an organ pipe and slightly increases acoustic transmission at these frequencies. Some animals can move their pinnae to orientate them towards a sound. In humans, with a relatively static pinna, the acoustic shielding effect of the head between the two ears and the diffraction of the sound around the head provides the major clue for the brain to work out where a sound is coming from. Clues about the elevation of a sound source are also provided by the reflection of sounds from the curved surfaces of individual pinnae, which we learn to use by processing the auditory signals. 5 The middle ear The middle ear is a cavity interposed between the eardrum (the tympanic membrane that closes off the ear canal), and the membranous oval window that opens into the cochlea of the inner ear but retains the fluid within it (Figure 1). In construction, the middle ear on each side of the head contains the ossicular chain of three bones (the malleus, the incus, and the stapes, collectively called the ossicles), that connect the external and inner ear structures (Figure 5). The middle ear contains (moist) air: its communication with the pharynx through the Eustachian tube allows the pressure on either side of the eardrum to be equalized to atmospheric pressure, as most airline passengers know.
Details
-
File Typepdf
-
Upload Time-
-
Content LanguagesEnglish
-
Upload UserAnonymous/Not logged-in
-
File Pages13 Page
-
File Size-