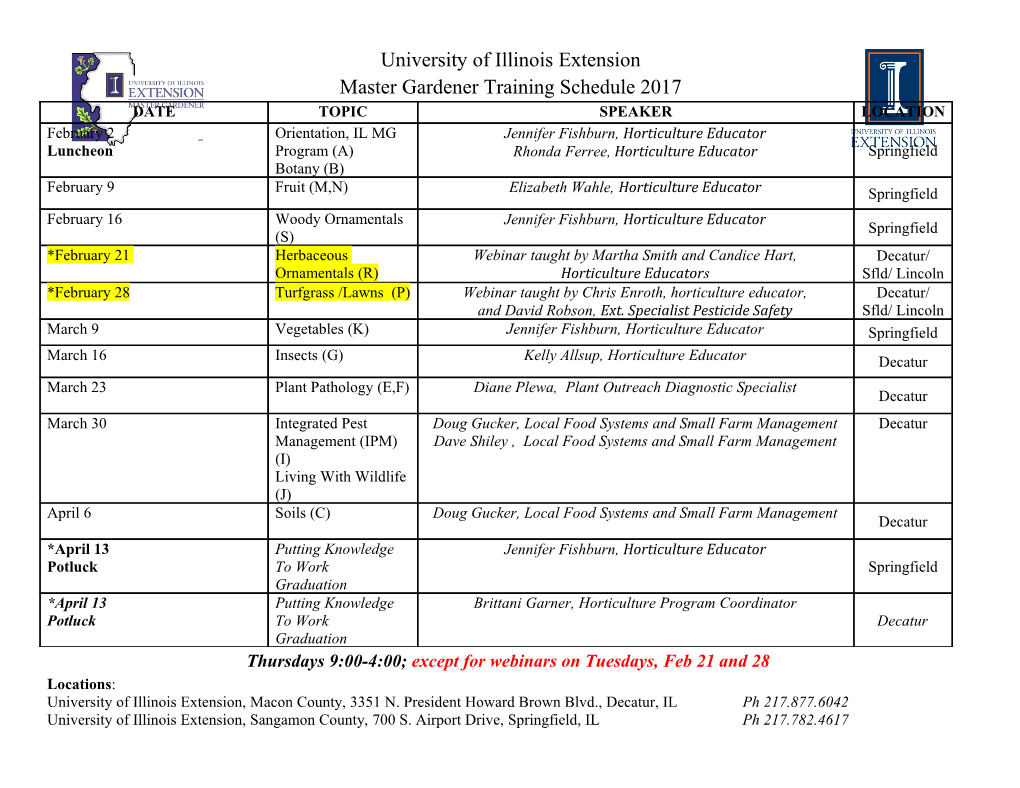
Synthetic Strategies and Parameters UNIT 3.3 Involved in the Synthesis of Oligodeoxyribonucleotides According to the Phosphoramidite Method The study of nucleic acids has, over the years, strategies for the preparation of oligodeoxyri- driven the development of fundamental method- bonucleotides. Milestones that led to the dis- ologies necessary for the examination of their covery of the phosphoramidite method for oli- structure and chemistry. The ability to produce godeoxyribonucleotide synthesis are chrono- substantial quantities of sequence-defined syn- logically reported. Alternate strategies to the thetic DNA has been invaluable to nucleic acid preparation of deoxyribonucleoside phos- research. Synthetic oligonucleotides have facili- phoramidites are then described to underscore tated structural investigations and provided a bet- the versatility with which these synthons can ter understanding of the interactions between be obtained. The mechanisms of deoxyribonu- nucleic acids and, for example, binding and/or cleoside phosphoramidite activation and the modifying proteins. In addition, synthetic oli- factors affecting condensation rates are dis- godeoxyribonucleotides have been extensively cussed along with the importance of “capping” applied to the preparation of primers for enzy- and oxidation reactions toward defining opti- matic synthesis, amplification, and DNA se- mal performance conditions for oligonu- quencing. They have also been commonly used cleotide synthesis. Finally, alternate phos- in site-directed mutagenesis experiments and as phoramidite methods to oligodeoxyribonu- hybridization probes for diagnostic purposes. cleotide synthesis and additional deprotection More recently, modified synthetic oligonu- strategies are presented to demonstrate facile cleotides have been targeted to cellular messenger access to synthetic oligonucleotides for a vari- RNAs in an attempt to control gene expression ety of applications. This unit is intended to and develop therapeutic agents against various complement APPENDIX 3C on the synthesis and types of cancer and human infectious diseases purification of oligonucleotides. (Beaucage and Iyer, 1993; Crooke and Bennett, 1996). On this basis, the availability of synthetic ACCOUNTS OF CHEMICAL oligonucleotides has undoubtedly paved the way RESEARCH IN DNA to the biotechnology revolution. OLIGONUCLEOTIDE SYNTHESIS The basic strategy in oligonucleotide syn- A major advance in the chemical synthesis thesis resembles that of the stepwise synthesis of oligodeoxyribonucleotides was accom- of polypeptides. Typically, a functionally pro- plished in the mid-1970s through the develop- tected nucleotide monomer is linked to a grow- ment of the “phosphite triester” method ing oligonucleotide chain that is then chemose- (Letsinger and Lunsford, 1976). This approach lectively deprotected and allowed to couple relied on the use of P(III) chemistry and en- with the next nucleotide monomer of the de- tailed the reaction of 5′-O-phenoxyacetyl sired sequence. Although the coupling of nu- thymidine S.1 with 2,2,2-trichloroethyl phos- cleotide monomers was traditionally carried phorodichloridite S.2 to generate the corre- out in solution according to the phosphodiester sponding deoxyribonucleoside-3′-O-phos- (Khorana, 1968) and phosphotriester (Letsin- phorochloridite S.3 and variable amounts of ger and Ogilvie, 1969) methods, these strate- (3′→3′)-dinucleoside phosphite triester S.4 gies ultimately culminated in the development (Fig. 3.3.1). Addition of 3′-O-mono- of modern automated techniques using the methoxytrityl thymidine to the reaction mix- phosphoramidite method (Beaucage and ture gave, after treatment with aqueous iodine, Caruthers, 1981; Caruthers et al., 1987a). the dinucleoside phosphate triester S.5 along Given the major impact the phos- with the symmetrical (3′→3′)- and (5′→5′)- phoramidite approach has had on the synthesis dinucleoside phosphate triesters S.6 and S.7, of oligonucleotides since its inception in the respectively. The formation of S.7 presumably early 1980s, this commentary provides an over- resulted from the reaction of 3′-O- Synthesis of view of the parameters affecting the perform- monomethoxytrityl thymidine with unreacted Unmodified ance of the method and a number of compatible S.2. It should be noted that the phosphite triester Oligonucleotides Contributed by Serge L. Beaucage and Marvin H. Caruthers 3.3.1 Current Protocols in Nucleic Acid Chemistry (2000) 3.3.1-3.3.20 Copyright © 2000 by John Wiley & Sons, Inc. PhOCH COO Thy 2 O Thy Thy PhOCH2COO Cl CCH OPCl PhOCH COO O 3 2 2 2 O 2 O P OH 2,6-lutidine/THF O Cl3CCH2O O P 1 Cl OCH CCl 2 3 O PhOCH2COO Thy 3 4 Thy PhOCH COO Thy Thy MMTrO HO 2 PhOCH2COO O O O 1. O O MMTrO O O Thy O POCH2CCl3 O P OCH2CCl3 O P OCH2CCl3 2. I /H O O Thy O O Thy 2 2 O O O MMTrO PhOCH2COO Thy MMTrO MMTr, 4-methoxytrityl 567 Ph, phenyl Figure 3.3.1 The phosphite triester method to oligodeoxyribonucleotide synthesis. approach to oligonucleotide synthesis was re- and inert atmosphere. Although nucleoside markably rapid; the preparation of S.5 was chlorophosphites/tetrazolides led to the rapid complete within 1 hr. In the mid-1970s, the and efficient preparation of DNA oligonu- formation of internucleotide linkages with such cleotides on silica supports, the technical hard- kinetics was unmatched. ship associated with handling these synthons Later, the deoxyribonucleoside chloro- impeded automation of oligodeoxyribonu- phosphite S.8 (Fig. 3.3.2) was synthesized and cleotide syntheses. reacted with deoxyribonucleosides covalently These problems vanished when deoxyri- attached to a silica support (S.9) through a bonucleoside phosphoramidites were devel- 3′-O-succinate linkage (Matteucci and Caruth- oped in the early 1980s (Beaucage and Caruth- ers, 1981; see also UNITS 3.1 and 3.2). The ers, 1981). These synthons were stable to air dinucleoside phosphate triester S.10 was pro- oxidation and hydrolysis under normal labora- duced in yields > 90%. Addition of 1H-tetrazole tory conditions but readily activated under mild to chlorophosphite S.8 significantly improved acidic conditions to form internucleotide link- condensation rates and coupling yields. The ages in near-quantitative yields. Because of application of nucleoside chlorophosphites or these properties, deoxyribonucleoside phos- the corresponding tetrazolides to solid-phase phoramidites have been conducive to auto- synthesis of oligonucleotides was, however, mated oligonucleotide synthesis on polymeric tedious. Because of inherent sensitivity to supports and are still the preferred synthons for moisture and air oxidation, the preparation of such syntheses. The preparation of these re- these reagents from reactive bifunctional agents was straightforward and involved the phosphitylating agents had to be performed at reaction of protected deoxyribonucleosides, −78°C under rigorously anhydrous conditions such as S.11, with chloro-(N,N-dimethy- DMTrO B O B DMTrO HO B O O O I2/H2O O O POMe O O O B N P O P H Cl OMe O O O 8 9 N P O H Oligodeoxyribo- DMTr, 4,4'-dimethoxytrityl B, Thy or any N-protected nucleobase 10 nucleotide Me, methyl Synthesis Using P, solid support the Phosphor- amidite Method Figure 3.3.2 Application of the phosphite triester approach to solid-phase DNA synthesis. 3.3.2 Current Protocols in Nucleic Acid Chemistry Cl DNA synthesis (Adams et al., 1983; McBride P DMTrO B Me N OMe O DMTrO B 2 and Caruthers, 1983). For instance, the de- O 12 O oxyribonucleoside phosphoramidite S.16 (Fig. OH i-Pr2NEt P 3.3.5), unlike S.13, survived silica gel purifica- Me2N OMe 11 13 tion and, as a result, consistently showed sta- Et, ethyl bility in acetonitrile solutions for several days i-Pr, isopropyl without significant decomposition (Adams et al., 1983). This class of phosphoramidites en- Figure 3.3.3 Preparation of deoxyribonu- abled reliable oligodeoxyribonucleotide syn- cleoside phosphoramidite monomers. theses (51-mers) on controlled-pore glass (CPG). In the early 1980s, these 51-mers were lamino)methoxyphosphine S.12 and N,N-di- the largest DNA segments ever chemically syn- isopropylethylamine (Fig. 3.3.3). The rapid re- thesized. action afforded deoxyribonucleoside phos- One drawback to the use of S.16 in auto- phoramidites S.13 without the wasteful forma- mated oligonucleotide synthesis is the noxious tion of (3′→3′)-dinucleoside phosphite thiophenolate treatment required for postsyn- triesters. The phosphoramidites S.13 were iso- thesis removal of the methyl phosphate protect- lated by conventional laboratory techniques ing groups (Daub and van Tamelen, 1977). and stored as dry powders (Beaucage and Although demethylation of methyl phosphotri- Caruthers, 1981). esters can be effected by 2-mercaptobenzothia- The phosphoramidite method is distinctive zole (S.17; Andrus and Beaucage, 1988) or in that it enables the conversion of relatively disodium 2-carbamoyl-2-cyanoethylene-1,1- stable deoxyribonucleoside phosphoramidite dithiolate (S.18; Dahl et al., 1990) under odor- derivatives into highly reactive intermediates less conditions, this deprotection step was in- suitable for DNA oligonucleotide synthesis. convenient because it added to the time and For example, addition of 1H-tetrazole to the reagents needed for oligonucleotide processing phosphoramidite S.13 and 3′-O-levulinyl (Fig. 3.3.5). In an effort to simplify and shorten thymidine S.14 in dry acetonitrile generated the postsynthesis deprotection protocols, the de- dinucleoside phosphite triester S.15 (Fig. 3.3.4) oxyribonucleoside
Details
-
File Typepdf
-
Upload Time-
-
Content LanguagesEnglish
-
Upload UserAnonymous/Not logged-in
-
File Pages20 Page
-
File Size-